ABOVE: © ISTOCK.COM, Kseniia Glazkova
Epigenetic changes alter the way genes are expressed throughout an organism’s life. These tweaks are then wiped from the genomes of reproductive cells, giving offspring a clean start—or so it seemed. Now, new evidence has emerged that epigenetic changes can be transmitted across multiple generations, despite the wipe. In a study published February 7 in Cell, a group of scientists tracked an engineered epigenetic mutation across four generations of lab-bred mice, finding evidence of the alteration in each of the subsequent generations. These alterations seemingly resurfaced even after the epigenetic wipe. The authors claim it is the first experimental evidence for transgenerational epigenetic inheritance using methylation-edited mice.
“It’s kind of a dream experiment,” says Patrick Allard, an environmental epigeneticist at the University of California, Los Angeles, who was not involved in the research. “And the results are both fascinating and confusing.”
Until the 1990s, it was generally assumed that any changes to genetic expression were the result of changes to the DNA sequence. That all changed with the advent of the field of epigenetics, which proved that environmental and behavioral factors can induce regulators to turn genes on or off, changing their expression without changing the DNA sequence itself. The most common mechanism through which different regulators can cause epigenetic change is DNA methylation. Through methylation, a molecule known as a methyl group attaches to DNA and signals to the rest of the cell whether that portion of the DNA should be turned on or off, thereby changing that DNA segment's effect on the resulting phenotype. According to Allard, an average of 70 percent of the genome is methylated, but until recently scientists have refused to believe that the changes to an organism’s methylation pattern pass on to their offspring. He says this disbelief stems from the fact that all but 10 percent of the methyl attachments on the genome of an embryo’s germ cells get wiped away before those cells give rise to the gametes. This process, which happens at least twice in germ cells before they go on to become gametes, creates what scientists in the field call a blank slate.
With so much of the inherited methylation pattern erased, Allard says it was initially hard to imagine that any changes seen in the parents could pass on to their children. “It really set the stage that there’s no environmentally negated inheritance that’s possible because you have this blank slate,” he explains.
See “Epigenetics May Remember Ancestors’ Mutations”
However, many scientists still believed epigenetic inheritance was happening; they just needed a better way to study the process. Editing the methyl tags artificially could give scientists control over which genes are expressed and open up new avenues to study the inheritance of epigenetic traits across generations. However, according to study coauthor Yuta Takahashi, an epigeneticist and senior scientist at biotechnology company Altos Labs, the technology to do this was limited until 2017, when he and his colleagues presented a method through which they used CRISPR Cas-9 to insert and modify methylation patterns in the genome of a human stem cell. With this new technology in hand, Takahashi could begin his research on the inheritance of epigenetic changes.
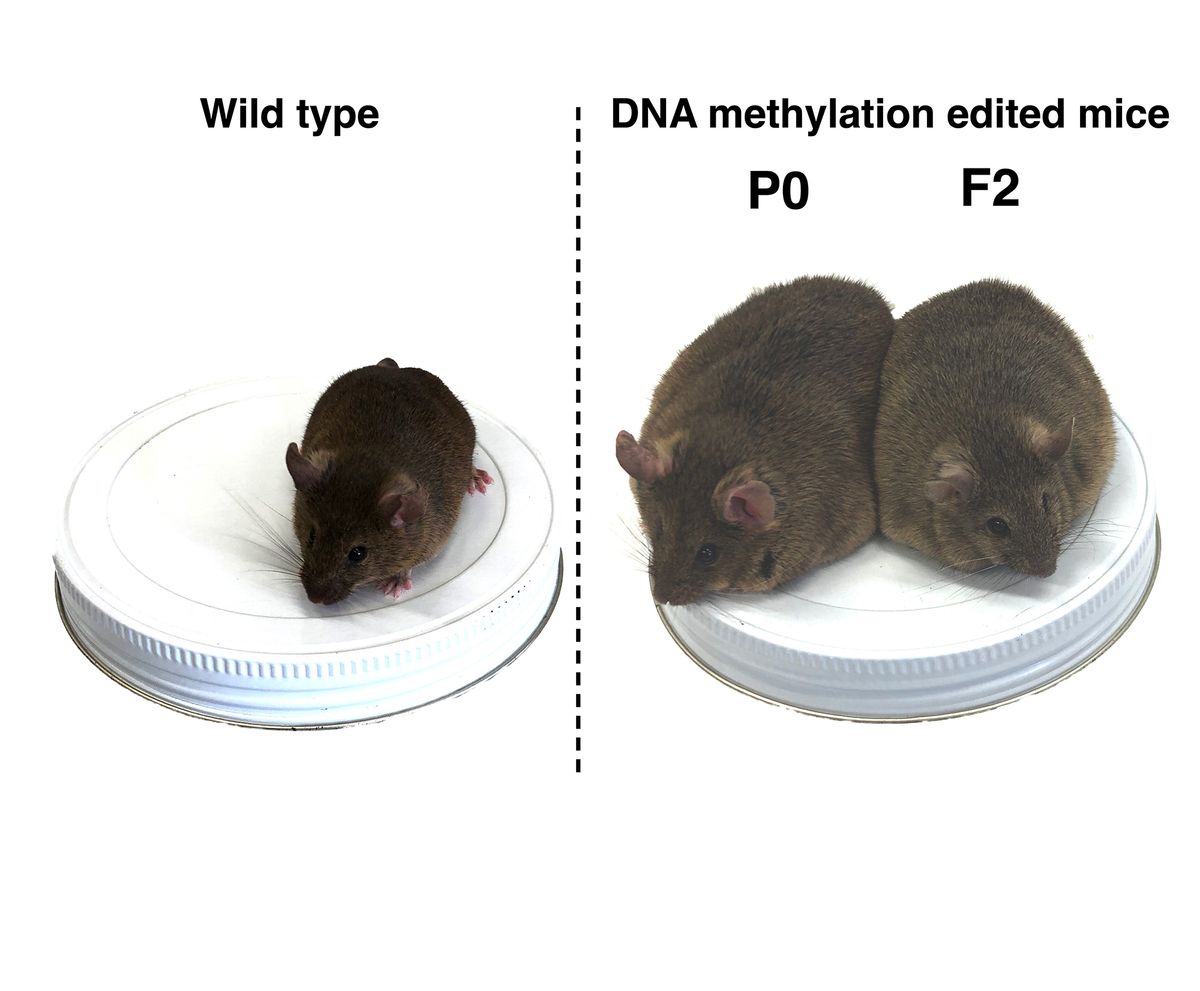
Using their DNA methylation editing technology, the scientists silenced two genes associated with metabolism (of interest due to their connection to diseases such as diabetes and obesity) in mouse stem cells. They then injected those stem cells into mouse embryos, placed the embryos into surrogate mothers and observed the mice from birth to ten months of age. As they’d hoped, the mice with epigenetically modified cells exhibited phenotypic changes; they became more obese than control mice and had higher cholesterol levels.
They then bred a male modified mouse to a female mouse from an unrelated strain. They collected DNA samples from the 12 resulting offspring to determine which had inherited the modified parent mouse’s alleles. They then picked one male and one female offspring mouse that had inherited the modified allele and bred each of them to mice from the same unrelated strain, continuing this process for four generations. After purifying and analyzing the methylation patterns of the samples, they found that all offspring mice throughout the four generations who inherited the modified allele displayed the inhibited methylation pattern, no matter if a female or male edited mouse was the parent. The phenotypic changes persisted as well; mice with the inhibited methylation pattern in the subsequent generations continued to be obese compared to controls and had high cholesterol levels.
“This is exactly what the field needed,” Allard says. “We needed to create a dedicated change in the epigenome and really carefully monitor it across generations through developmental stages.”
See “Does Human Epigenetic Inheritance Deserve a Closer Look?”
To Allard, the most confusing and fascinating result of the study was the way in which the epigenetic changes presented during development. In the DNA taken from the mice’s germ cells, Takahashi and his colleagues saw the methylation erasure scientists had pointed to before. But later on in the embryo’s development, the old methylation patterns in the genes they’d modified resurfaced and stuck around as the mouse matured. This indicated that there was some sort of system that somehow retained a memory of these lost patterns, a finding that came as a surprise to many epigenetics researchers who discussed it online.
“There were so many questions of ‘could something resist that epigenetic reprogramming, that blank slate?’” says Allard. “And the answer is, at least for these [genes], that they don’t need to resist it. You can have your blank slate and still be recreated.”
Takahashi emphasizes that they still have a lot of research to do. “It’s very exciting, but when you face a very great result, you have to confirm these results.” It’s possible, for example, that the ability of this epigenetic modification to pass on could be entirely due to the way it was engineered, or that the genes they chose happened to be special exceptions. Allard also hopes they can get a better understanding of the erase-and-replace methylation patterns. “What does that molecular memory look like?” he asks. “What is really the mechanism?”
See “Do Epigenetic Changes Influence Evolution?”
If their work turns out to be reproducible, Takahashi says the next step is to take the research to humans, and determine once and for all whether transgenerational epigenetic inheritance occurs in us. If so, he stresses there could be profound implications for the way we understand and treat inherited diseases. Allard stresses that epigenetic research in humans is incredibly difficult because of our long generation time, and also brings up a lot of moral and ethical questions. “I don’t know how we would do this experiment in humans,” he says. “But if [the result] is validated in the mouse, it would form a very strong foundation” of results.