Article reviewed by Bruno Chue, Senior Fluorescence Microscopy Technician at the University of Toronto Scarborough.
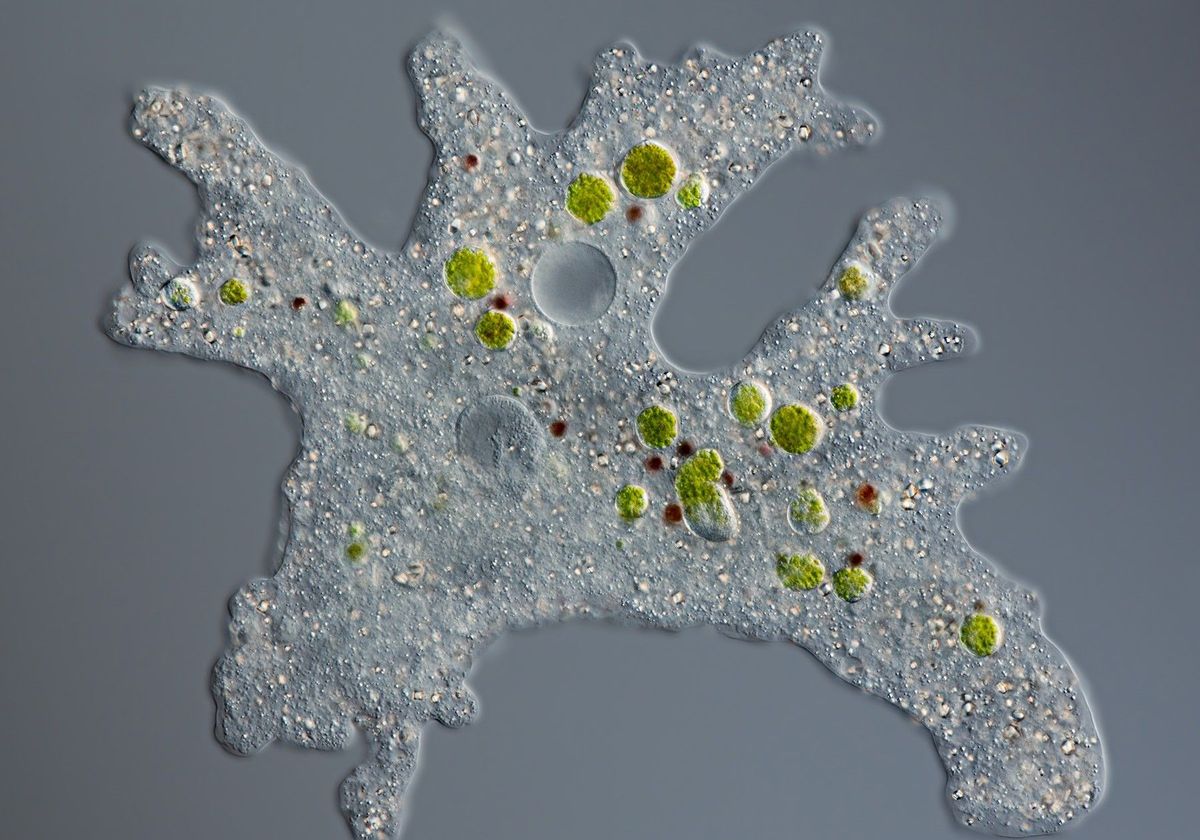
Stay up to date on the latest science with Brush Up Summaries.
What Is Live Cell Imaging?
Live cell imaging is an analytical microscopy-based technique that researchers use to observe living cells over several seconds, hours, or even days.1 Through time-lapse microscopy, scientists track proteins, organelles, individual cells, or cell populations during dynamic processes, such as phagocytosis, wound healing, or differentiation.
Advantages and disadvantages of live cell imaging
Compared to fixed cell imaging that captures static snapshots of potentially fixation-perturbed processes, live cell imaging allows researchers to follow cells closer to their native state in vivo, uncovering new insights into cellular events as they happen.2 Researchers can observe these processes using unlabeled cells or by employing fluorescent dyes and antibodies to label specific biomolecules or organelles without fixation.3 Additionally, scientists can induce cells to express fluorophore-conjugated proteins including green fluorescent protein (GFP) or red fluorescent protein (RFP). Researchers have not only employed live cell imaging to observe individual cells in culture, but also to follow cells within organoids or living animals including mice and zebrafish.4
Table 1: Comparing live and fixed cell imaging3,5
Live Cell Imaging | Fixed Cell Imaging | |
Investigating cellular processes | Captures a dynamic process in real time | Captures snapshots of various parts of a process |
Observing rare events | More likely | Unlikely |
Requires specialized equipment to maintain cellular health | Yes | No |
Affected by photobleaching | Yes | Yes |
Affected by phototoxicity | Yes | No |
Data file size | Large | Small |
Level of difficulty | Very challenging | Relatively simple |
Microscopy Methods for Live Cell Imaging
Scientists categorize most imaging methods into two major types: label-free and fluorescence microscopy. However, these techniques do not exist in isolation, as the majority of fluorescence microscopes also come equipped with the capability to capture label-free images.
Brightfield microscopy
Brightfield microscopy is a common and basic label-free imaging technique that fully illuminates cells with white light.6 Dense areas within specimens absorb some of this light and researchers observe the light that passes through the sample, called the transmitted light, through the microscope’s eyepiece or in images captured by a camera. While this technique is simple to employ and generates enough contrast between the specimen and background for some samples, it is difficult for scientists to observe cellular structures with brightfield illumination as cells are translucent.7 Consequently, researchers have developed new label-free imaging techniques to improve image contrast.
Phase contrast microscopy
Phase contrast microscopy is a label-free imaging method with two forms: positive and negative phase contrast microscopy.7 Light rays that pass through various regions of a cell experience changes in speed, which are known as phase shifts. Using phase rings, a positive phase contrast microscope amplifies the phase differences between the deviated light and the undeviated background light rays that have not encountered the specimen, while a negative phase contrast microscope eliminates the phase shifts between these rays. When the microscope focuses the deviated and background light waves on the same plane, the waves interfere destructively with positive phase contrast microscopy or constructively with negative phase contrast microscopy, which changes the amplitude of the resulting wave.7 This alters the light wave’s intensity, thereby producing contrast for better cell detection.
DIC microscopy
Differential interference contrast (DIC) microscopy is another label-free imaging method that researchers use to increase contrast. This technique uses a polarizer and prism to produce two orthogonal beams of polarized light that pass through the cells or surrounding environment and undergo phase shifts relative to one another.8 When a second prism and polarizer reunite the light beams and force them to vibrate in the same plane, they destructively and constructively interfere with each other and combine into a single beam.9 Differences in the amplitudes of the resulting waves lead to variations in light intensity, thereby generating contrast.
Widefield fluorescence microscopy
Widefield fluorescence microscopy is a basic fluorescence imaging method. Similar to brightfield microscopes, a widefield fluorescence microscope exposes the entire specimen to the light.10 In this case, a specific wavelength of light excites a fluorophore, which emits a photon at a longer wavelength. Researchers then observe this emitted light through the eyepiece or in images obtained by the camera.10 Although the technique is relatively simple to use, widefield fluorescence microscopy illuminates all focal planes of the cell and the resulting out-of-focus emission light reduces the image resolution by masking the fluorophore’s signal from the chosen focal plane. Thus, scientists developed several imaging techniques to only capture the emitted light from a single focal plane.
Confocal microscopy
Confocal microscopy is a fluorescence imaging technique with two major forms: laser-scanning and spinning disk confocal microscopy.10 Both types use a laser beam to excite the fluorophores in a small area of the sample and pinhole apertures to reduce the detection of out-of-focus light. This allows researchers to optically section the cells and capture high-resolution images of a single focal plane.10 A laser-scanning confocal microscope uses oscillating mirrors to scan the laser beam point by point across the sample horizontally from left to right and line by line, which is called a raster scan. In contrast, a spinning disk confocal microscope employs a rotating disk with numerous pinholes to scan multiple points on the chosen focal plane simultaneously.
Lattice light-sheet microscopy
Lattice light-sheet microscopy is a fluorescence imaging method, which uses a two-dimensional grid pattern of laser light to illuminate a thin section of the sample.11 As the microscope only excites fluorophores close to a single focal plane, this technique reduces out-of-focus light and increases image resolution.
Two-photon excitation microscopy
Two-photon excitation microscopy is a fluorescence imaging technique that uses a pulsed laser beam to simultaneously direct two light waves toward the same point on the focal plane.12 While a single photon at a specific wavelength excites the fluorophore in other fluorescence microscopy methods, in this technique each photon’s individual wavelength can be twice as long because they excite the fluorophore together. This means that researchers can employ infrared illumination as their excitation light, which allows for deeper tissue penetration and reduced toxicity.12 As a result of these advantages, scientists use two-photon excitation microscopy to image cells in vitro and in vivo, such as observing cells deep within a mouse retina.13
Considerations for Live Cell Imaging
Live cell imaging is a challenging technique to master and requires researchers to consider several factors that can affect the success of the experiment.
Maintaining an ideal environment
To ensure researchers capture biologically relevant data, cells must remain healthy throughout the imaging period. This requires tight control of the cells’ environment including the temperature, osmolarity, and pH, where scientists regulate these parameters through an environmental control system.5 Researchers typically employ an inverted microscope for live cell imaging, where the objective lens is below the sample, which allows the cells to remain within the culture medium during imaging. Additionally, scientists use a stage-top incubator or heated enclosure that surrounds the entire microscope to maintain the specimen’s optimal temperature and ensure cellular processes are running as they would in vivo.5 These specialized chambers also allow the researchers to keep the cells in a highly humid environment to minimize evaporation of the culture medium during imaging, which would concentrate solutes such as ions and waste products within the fluid and stress the cells. Scientists often use culture medium with bicarbonate and chamber-injected carbon dioxide to buffer any changes to the sample’s pH.5
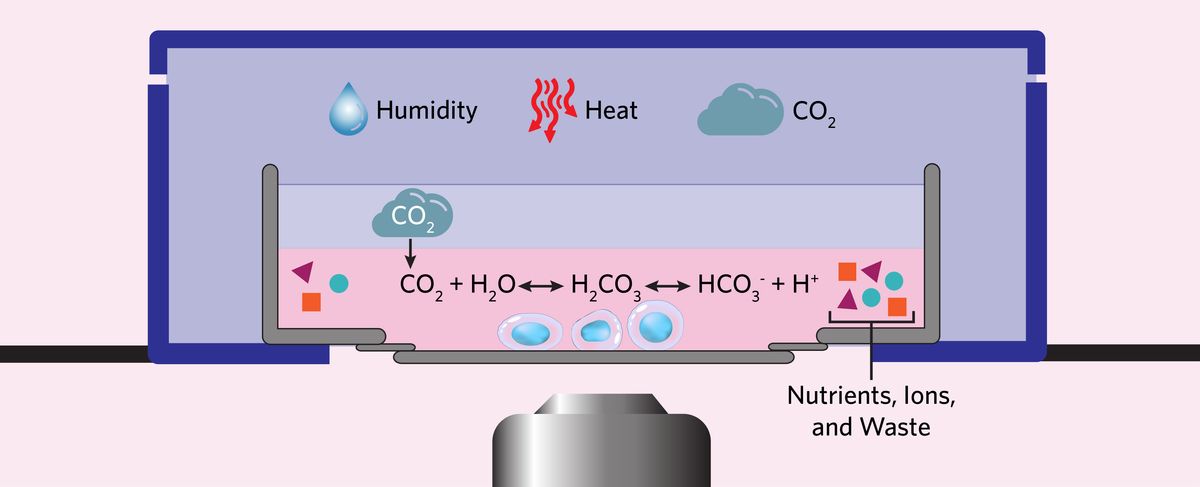
Choosing appropriate fluorophores
Scientists must carefully choose the fluorophores they plan to employ for specific live cell imaging experiments because not all fluorophores are ideal for every scenario.5 For example, GFP’s fluorescence becomes dampened or quenched in acidic conditions, such as within the cell’s lysosomes, and thus, researchers are unable to use most GFP-tagged lysosomal proteins. To observe multiple fluorescently-labeled biomarkers simultaneously, scientists must also avoid using fluorophores that have similar excitation and emission spectra.10 Moreover, researchers employ fluorophores whose fluorescent signal diminishes quickly for short-term imaging, while they can use other more photostable fluorescent molecules to follow cellular processes over multiple days. Besides traditional fluorophores, scientists also use quantum dots for live cell imaging, which are inorganic fluorescent nanocrystals that are brighter and more photostable than other fluorophores.14
Preventing photobleaching and phototoxicity
Scientists must determine the ideal image acquisition settings required for live cell imaging.14 Some experiments may need higher-intensity excitation light, longer exposure times, shorter time periods between images, or all three depending on fluorophore abundance and the speed of the cellular process. However, employing these settings has consequences. For instance, repetitive exposure to high-intensity light leads to photobleaching, where the fluorophores become increasingly damaged, reducing the fluorescent signal over time.5 Besides decreasing the signal, this experimental setup can also harm or kill the cells, which is a phenomenon known as phototoxicity.15 Researchers must find the best settings, which strike a balance between keeping the cells healthy and obtaining the high-quality images they require.
- Cuny AP, et al. Live cell microscopy: From image to insight. Biophys Rev. 2022;3(2):021302.
- Stephens DJ, Allan VJ. Light microscopy techniques for live cell imaging. Science. 2003;300(5616):82-86.
- Cole R. Live-cell imaging. Cell Adhes Migr. 2014;8(5):452-459.
- Weigert R, et al. Imaging cell biology in live animals: Ready for prime time. J Cell Biol. 2013;201(7):969-979.
- Ettinger A, Wittmann T. Chapter 5 - Fluorescence live cell imaging. In: Methods in Cell Biology. Vol 123. Academic Press; 2014:77-94.
- Rohde M. 4 - Microscopy. In: Methods in Microbiology. Vol 38. Academic Press; 2011:61-100.
- Sanderson JB. Phase contrast microscopy. In: Encyclopedia of Life Sciences. John Wiley & Sons, Ltd; 2001:1-4.
- Salmon ED, Tran P. High-resolution video-enhanced differential interference contrast light microscopy. In: Methods in Cell Biology. Vol 81. Academic Press; 2007:335-364.
- Keller HE. Contrast enhancement in light microscopy. Curr Protoc Cytom. 2001;00(1):2.1.1-2.1.11.
- Sanderson MJ, et al. Fluorescence microscopy. Cold Spring Harb Protoc. 2014;2014(10):pdb.top071795.
- Chen BC, et al. Lattice light-sheet microscopy: Imaging molecules to embryos at high spatiotemporal resolution. Science. 2014;346(6208):1257998.
- Benninger RKP, Piston DW. Two-photon excitation microscopy for the study of living cells and tissues. Curr Protoc Cell Biol. 2013;Chapter 4:4.11.1-4.11.24.
- Palczewska G, et al. Noninvasive two-photon microscopy imaging of mouse retina and retinal pigment epithelium. In: Retinal Degeneration: Methods and Protocols. Springer; 2019:333-343.
- Jensen EC. Overview of live-cell imaging: Requirements and methods used. Anat Rec. 2012;296(1):1-8.
- Icha J, et al. Phototoxicity in live fluorescence microscopy, and how to avoid it. BioEssays. 2017;39(8).
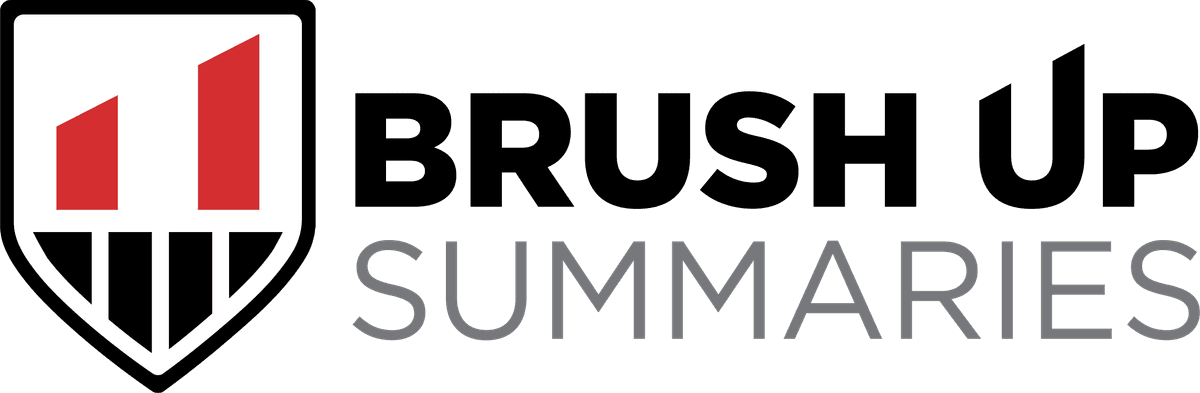