ABOVE: Human cells repurpose an enzymatic seamstress to repair CRISPR-guided cuts in RNA. ©ISTOCK, BlackJack3D
Like the find-and-replace feature of a word processor, CRISPR-based engineering allows scientists to quickly and efficiently edit DNA sequences with base pair precision. Lauded as the great democratizer of gene editing, CRISPR technologies have advanced disease modeling, functional genomics, and gene therapies.1 However, similar tools for RNA editing remain limited.
At first glance, it may seem counterintuitive to edit a transient molecule like RNA when tools exist for targeting the root of the problem: the DNA. However, DNA editing is not without its risks, such as off-target mutations, and its permanence is limiting when a temporary fix is desirable, such as developmental interventions or short-term cancer treatments. “This is where RNA editing might be really game changing,” said Anna Nemudraia, a molecular biologist at Montana State University (MSU).
In a paper published in Science, Nemudraia and colleagues used a unique CRISPR system to cleave RNA in human cells and identified the repair mechanism that cells use to stich the molecule back together.2 Using cell models, the team showed that their technology snipped out a disease-causing mutation common in patients with cystic fibrosis. The findings highlight the therapeutic potential of programmable RNA editing, but also provide researchers with a tool to study more fundamental questions in RNA biology—like how, and why, do human cells repair RNA?
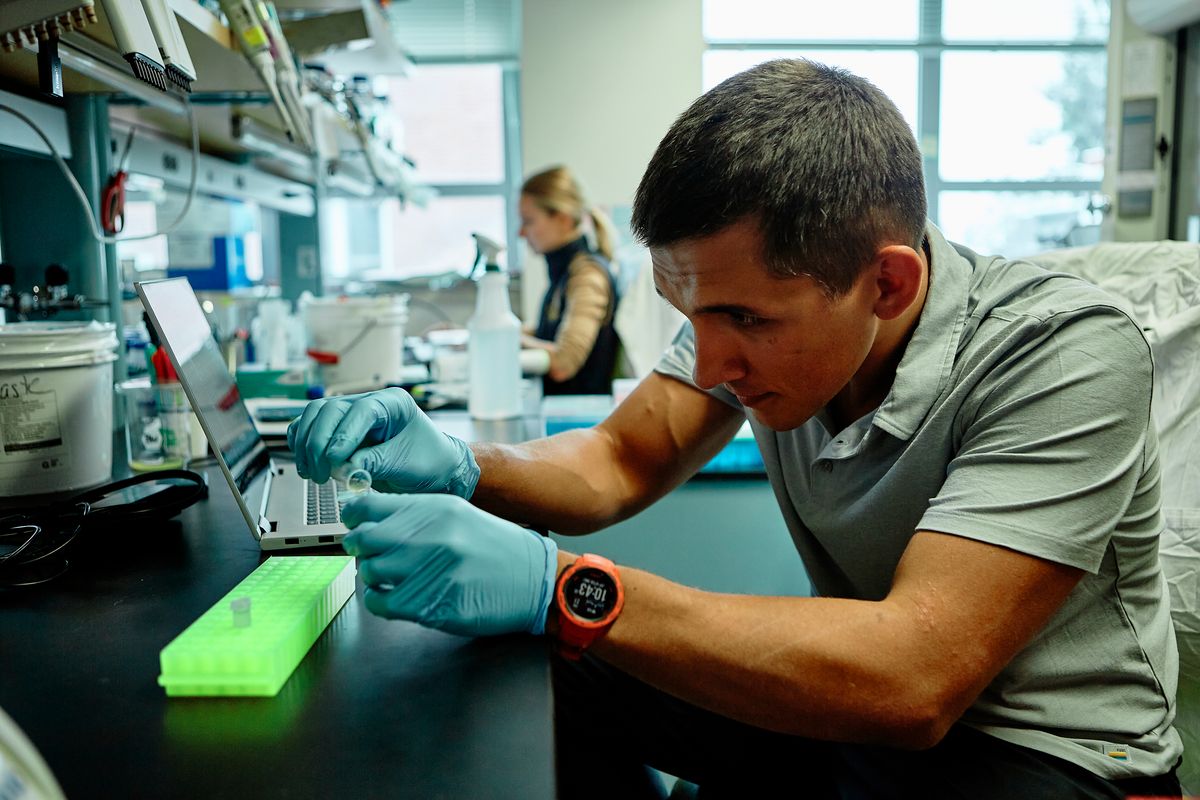
“The paper overall is very creative,” said David Cox, a geneticist at Stanford University who was not involved in the study. “[This technology] is going to add to the arsenal of RNA editing tools that are available, especially for mammalian cells.”
“[The research] was inspired by an observation that we made during the COVID-19 pandemic,” said Artem Nemudryi, a geneticist at MSU and coauthor on the paper. Back in 2020, Nemudryi and Nemudraia were studying CRISPR systems in Blake Wiedenheft’s laboratory, but they switched gears to study mutations in the SARS-CoV-2 virus, joining the global effort to understand how the genome of this RNA virus changed over time. While exploring mutations in ORF7a, a viral accessory protein that antagonizes antiviral host responses, they discovered a rare strain with a 115-nucleotide-long deletion in the virus’s 30,000-nucleotide-long RNA genome.3
As treatments and vaccines became available, the researchers had time to wonder about how RNA viruses repair deletions in their genome, and they started looking for tools that allowed them to directly induce cuts into viral RNA. CRISPR-guided endonucleases were an obvious route, but for RNA, the options were few and imperfect. Cas13 proved useful for RNA knockdown, but it caused collateral damage to non-target RNA. Although the nuclease-inactivated mutant dCas13 did not lead to off-target cleavage, its editing capabilities are still limited—they cannot induce programmable deletions at a specific location in the RNA sequence.4
“Our motivation for this work was to unlock other possibilities for RNA editing,” said Nemudryi; he was searching for a system that could substitute or remove chunks of nucleic acids in the viral genome. The research team landed on a type III CRISPR complex, which, like Cas13, targets RNA, but does so in an odd—or rather, even—manner: it cleaves the RNA in six-nucleotide increments.5 “The type III CRISPR system is very sophisticated and unique,” said Nemudraia.
In a study published last year in Science Advances, the researchers used this CRISPR ribonuclease to make six-nucleotide-interval snips in viral RNA for programmed deletions or insertions in the genome.6 After this viral success, the researchers wondered whether this type III CRISPR system could also edit human RNA. To test this, they targeted the system towards two transcripts that are highly expressed in the HEK293T human cell line and then sequenced the RNA.
“The most exciting moment in this paper was when we got the first sequence in,” said Nemudryi. The researchers were looking at the alignment of the sequencing reads when they noticed gaps that were consistent with the six-nucleotide cleavage pattern. “These sort of deletions of six, 12, or 18 [nucleotides], they don't happen at random—we knew at the time that there is [RNA] repair going on,” said Nemudryi.
When type III CRISPR complexes cleave RNA, they leave a specific chemical fingerprint at each of the two cut sites.7 In mammalian cells, endogenous splicing of transfer RNA causes the same marks, which the RNA ligase RTCB stitches back together.8 Knock down and overexpression experiments of RTCB helped confirm that this enzymatic seamstress was also repairing the damage inflicted by an exogenous actor, the CRISPR complex.
“As somebody who’s worked in the field of RNA and RNA engineering, it's pretty surprising that these [CRISPR-guided] RNA breaks are repaired,” said Cox. Since RNA is a transient molecule, the dogma is that a cell would clear damaged transcripts and synthesize new ones. “We are interested in the physiological role of this process,” said Nemudryi. "Do cells repair RNA and why do they do that?”
To explore the clinical utility of their technology, the researchers tested it in a cell model of cystic fibrosis that expressed mutation in the cystic fibrosis transmembrane conductance regulator (CFTR) transcript. The mutation, a premature stop codon, sets off the cell’s alarm bells once the transcript enters the cytoplasm, triggering its decay before it becomes a functional protein. Insufficient CFTR levels affect ion transport in the lungs and contribute to the development of cystic fibrosis. The researchers programmed a type III CRISPR to target the mutant CFTR transcripts. The CRISPR complex, which harbored a special tag that localized its expression to the cell’s nucleus, hunted down and edited faulty mRNA before it escaped into the cytoplasm.
Although the researchers demonstrated that their break-and-repair RNA editing tool snipped out the CFTR mutation, the efficiency was low, something the researchers noted is a common teething problem that all CRISPR editors go through early in their development. Furthermore, they noted that the current version of the type III CRISPR has a mind of its own, changing its cleavage pattern from target to target. For example, the complex may make three cuts and snip out 12 nucleotides in one target but induce four cuts to remove 18 nucleotides in another. With further tweaks to the system, they hope to wrangle the ribonuclease to achieve more precise and efficient RNA editing.
Nemudryi and Nemudraia, who have been working side by side at the bench for the last six years, will start their own research group at the University of Florida this fall, where they will continue these efforts.
“The beauty of this work is that it's not just a technology, but also we created a tool,” said Nemudraia, who hopes to use the CRISPR-guided cut-and-repair system to further explore RNA repair mechanisms.
- Wang JY, Doudna JA. CRISPR technology: A decade of genome editing is only the beginning. Science. 2023;379(6629):eadd8643.
- Nemudraia A, et al. Repair of CRISPR-guided RNA breaks enables site-specific RNA excision in human cells. Science. 2024;384(6697):808-814.
- Nemudryi A, et al. SARS-CoV-2 genomic surveillance identifies naturally occurring truncation of ORF7a that limits immune suppression. Cell Rep. 2021;35(9):109197.
- Cox DBT, et al. RNA editing with CRISPR-Cas13. Science. 2017;358(6366):1019-1027.
- Tamulaitis G, et al. Programmable RNA shredding by the type III-A CRISPR-Cas system of Streptococcus thermophilus. Mol Cell. 2014;56(4):506-517.
- Nemudryi A, et al. CRISPR-based engineering of RNA viruses. Sci Adv. 2023;9(37):eadj8277.
- Hale CR, et al. RNA-guided RNA cleavage by a CRISPR RNA-Cas protein complex. Cell. 2009;139(5):945-956.
- Popow J, et al. HSPC117 is the essential subunit of a human tRNA splicing ligase complex. Science. 2011;331(6018):760-764.