Stay up to date on the latest science with Brush Up Summaries.
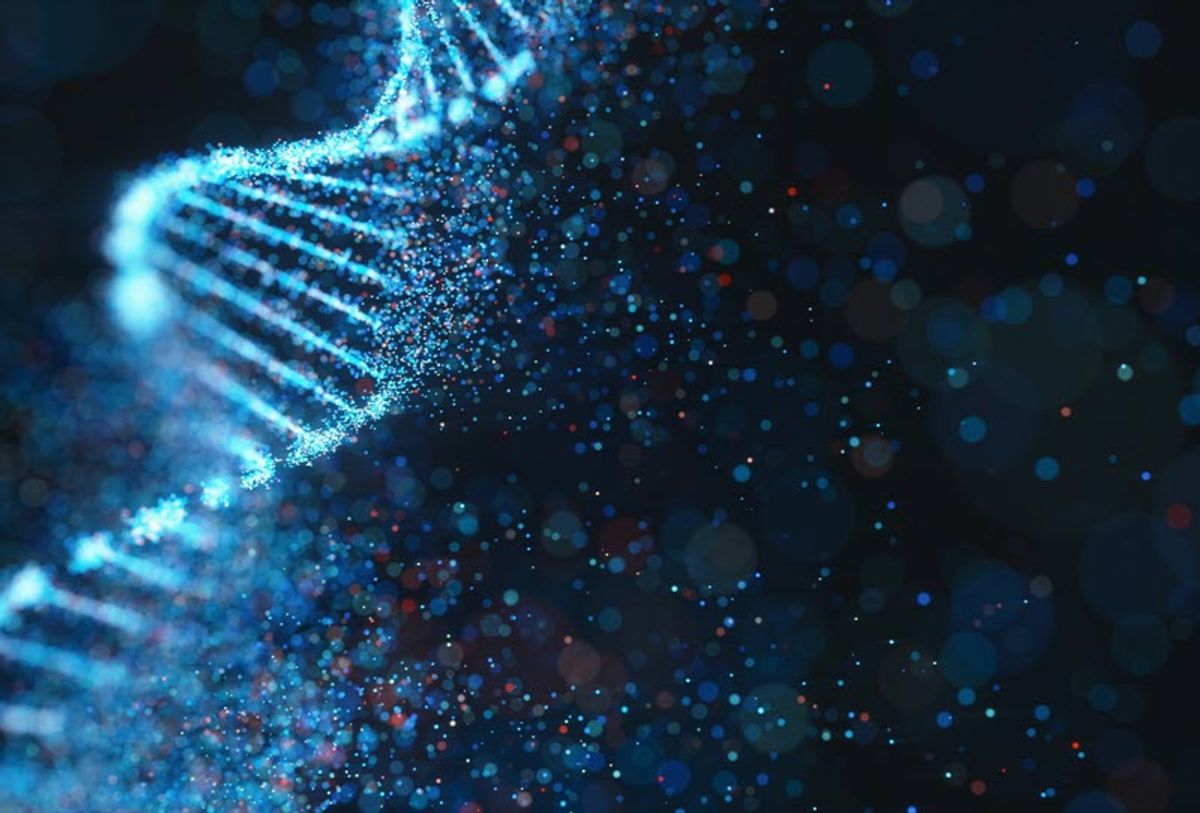
What Are Synthetic Genomes?
Synthetic genomes are genetic materials designed and developed by scientists to study biological systems of natural organisms.1 “I would define a synthetic genome as a genome of an organism in which its entire DNA content is being designed by scientists on the computer and then actually assembled piece by piece in the laboratory,” said Wes Robertson, a synthetic biologist at the Medical Research Council (MRC) Laboratory of Molecular Biology.
Researchers follow the design–build–test–learn cycle while developing synthetic genomes. It is an iterative process for constructing and improving functional synthetic chromosomes. To date, scientists have been able to create designer versions of the entire genomes for Escherichia coli and Mycoplasma mycoides, and a large part of the genome for Saccharomyces cerevisiae.2,5,7,9
The Rapid Evolution of Synthetic Genomics
Synthetic genomics is an emerging field that achieved many significant milestones in the last two decades.
Synthetic viruses
Scientists developed the first synthetic viral genome of the poliovirus (∼7.9 kb) in the early 2000s.3 This success paved the way for scientists to develop synthetic genomes for other microorganisms. Subsequently, researchers also synthesized an artificial bacteriophage φX174 to treat drug resistant bacterial infection.4
Minimal synthetic bacterial cell
In 2010, scientists at the J. Craig Venter Institute designed a synthetic Mycoplasma genome (JCVI-syn1.0) of one million base pairs, which supported cell division and growth.5 They named the first bacterium with a synthetic genome Synthia. A series of transposon mutagenesis experiments led to the development of JCVI-syn3.0, which contains the smallest genome of any self-replicating organism.6 Scientists crafted JCVI-syn3.0 using a design, build, and test (DBT) process. They removed genes and DNA segments that were not essential for cell division and identified seven genes that were absolutely necessary for the normal functioning of JCVI-syn3.0. This was a significant milestone in synthetic genomics as it is the first real minimal cell.
E. coli and yeast synthetic genomes
After synthetic Mycoplasma genome development, scientists designed partial and complete synthetic E. coli genomes, namely, rE. coli-57 and Syn61, respectively.2,7 The successful assembly of the four million base pair Syn61 genome also demonstrated the plasticity of the genetic code—cells could grow with only 61 codons as the blueprint for life. Subsequent research with this synthetic strain highlighted the promise of redesigning genomes from scratch, mainly by demonstrating emergent functions such as viral resistance and unnatural polymer synthesis.8
In 2011, researchers from different countries collaborated to start the Synthetic Yeast Genome Project (Sc2.0) with the aim to develop the first synthetic version of each Saccharomyces cerevisiae chromosome.9 This is an ongoing research project with the ultimate goal of assembling a yeast organism with designed features. The entire yeast genome is 12 Mb and each chromosome is around 1 Mb in size. In comparison to bacterial genomes, designing yeast synthetic genomes has been more challenging and time consuming, which could be attributed to the fact that a significant section of yeast chromosomes remains unannotated. Therefore, the removal of DNA sequences could lead to unwanted functional consequences.
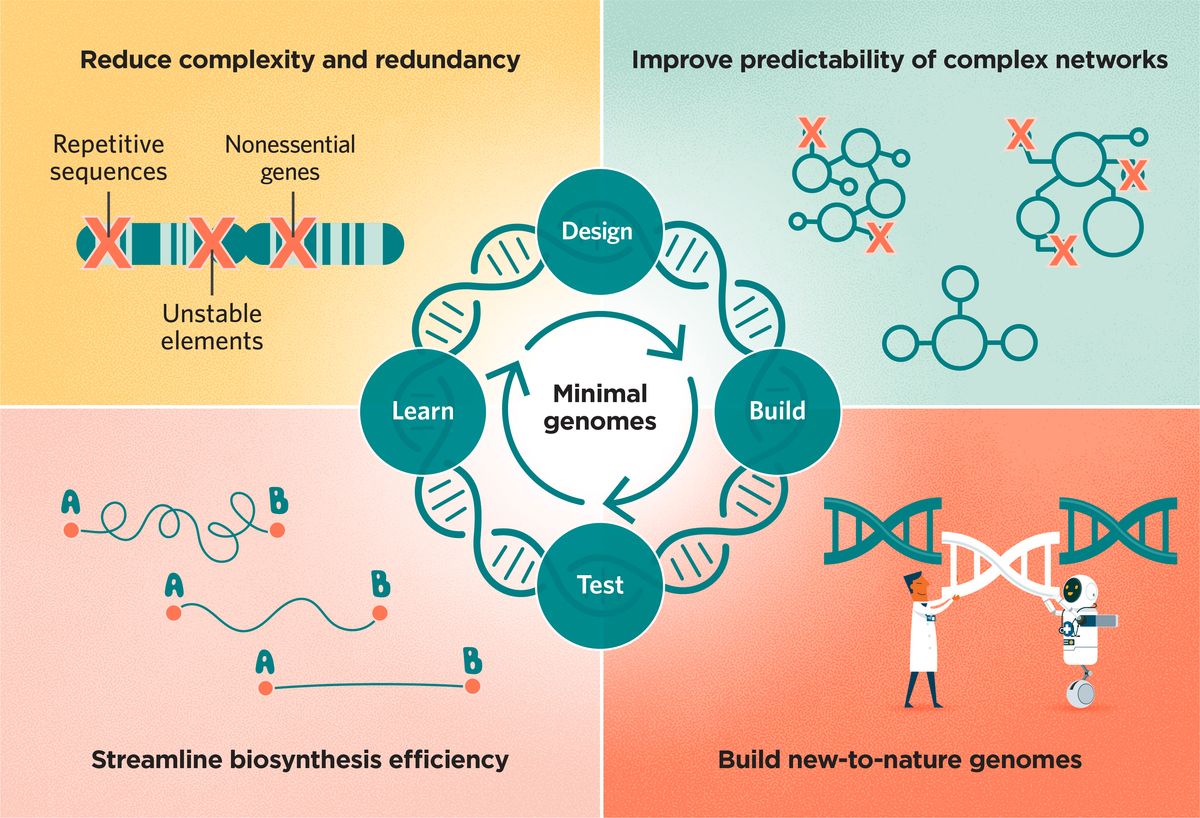
Modified from © Stock Images, Royalty-Free Images, Illustrations, Vectors and Videos - iStock , Stefan_Alfonso, Tasha Vector, DStarky, Natalya Chernyak, sorbetto; Designed by Ashleigh Campsall
Strategies to Design Synthetic Genomes
Scientists first aim to design genomes with minimal changes compared to the wild type to minimize the risk of failure. “The native microbe is used as a template and then you can make some manipulations,” said Robertson. These manipulations intend to introduce new functional features while meticulously balancing the native functions of replication, reproduction, and growth.
Genome construction involves three basic steps: oligonucleotide synthesis, genome assembly, and delivery of the synthetic genome into cells. Refactoring or streamlining is an acceptable strategy for designing synthetic genomes.2 This method involves disentangling genetic elements into stand-alone parts, which enables the assembling and testing of individual genetic segments for their functions. Typically, scientists employ this strategy to develop small sized and compact synthetic viral genomes such as the T7 bacteriophage.
While constructing synthetic bacterial genomes, researchers place emphasis on minimizing and re-functionalizing the native genomes.10 Reducing the genomic redundancy is a viable strategy because all DNA elements are not essential to carry out fundamental processes. For example, a bacterium might not require specific genetic features when grown under nutrient-enriched and stress-free conditions. Over the years, researchers reduced the size of the E. coli genome by 29% using various strategies, including multiple-deletion series to develop new strains with smaller genomes.11
In contrast to prokaryotes, eukaryote genomes are far more complex due to their larger size and complexities in functional regulations. For the sc2.0 yeast synthetic genome, scientists removed repeated sequences, such as subtelomeric repeats, retrotransposons, and pre-mRNA and pre-tRNA introns.9 The Synthetic Chromosome Recombination and Modification by LoxP-mediated Evolution (SCRaMbLE) system endowed yeast strains with semisynthetic genomes the ability to rearrange its genome in novel ways and to thus explore novel evolutionary trajectories. This system has been demonstrated to evolve strains with improved tolerance to variable stress factors such as heat, acetic acid, and ethanol.
Delivery of the synthetic genome into cells
Scientists test the designed genomes in living systems by introducing them into the host via one step delivery or stepwise substitution delivery depending on the genome size. The one step method is a straightforward process that involves direct transplantation of the complete synthetic genome in a recipient cell. Scientists used this method to transplant designed Mycoplasma mycoides genomes into an M. capricolum recipient cell to create new M. mycoides cells. The synthetic genome replaces the native genome and controls the new cell completely, which can also replicate continually. However, this method is not ideal for isolating and manipulating large sized DNA due to its fragility. Stepwise substitution overcomes this methodological shortcoming by iteratively substituting wild-type genome fragments with their synthetic counterparts. Scientists used this method to develop semisynthetic yeast genomes as well as the complete synthetic E. coli genome (Syn61).2
Challenges in designing synthetic genomes
Developing an entire genome from scratch is difficult. The large genomic size and functional complexities make this a particularly hard task. “Life is complex, and while we do have genome annotations for many microorganisms, we don’t necessarily understand what the full genome annotation is,” Robertson explained. Because of this, creating a new synthetic genome runs the risk of unintentionally changing or eliminating unannotated or partially annotated genes. For example, scientists conducted extensive research on short open reading frames, which are often not included in conventional genome annotation methods because of their small size or them being nested within parental open reading frames. Normally, these sequences are mistaken as a part of another gene, but these are independent genes that share the same DNA. “If you start redesigning a synthetic genome and changing the sequence of the parental gene, you’ll accidentally change the sequence of this other gene as well, which is an unintended consequence and that will affect biological function,” said Robertson.
Synthetic Genome Synthesis
Scientists construct synthetic genomes using the bottom up method or top down genome editing process.10 The bottom up method employs single stranded oligonucleotides as the starting material.2 Typically, high-throughput and automated oligo synthesis technologies enable a higher oligonucleotide production per day. The meticulous assembly of single-stranded oligonucleotides via in vitro and in vivo methods aids the synthesis of designed DNA sequences. For example, researchers first developed the Gibson assembly technique for the Mycoplasma project. This method assembles smaller DNA pieces based on defined overlaps to develop a larger piece of DNA.
The top down genome editing process is a genome synthesis method that uses tools such as zinc finger nucleases, CRISPR-Cas9 systems, and transcription-activator-like effector nucleases to develop designer genomes with favorable features.12
Both bottom up and top down genome editing methods have distinct advantages and limitations. For instance, bottom up synthesis methods enable the insertion of all the designed genomic elements at once. This approach is required for closely spaced genomic alterations positioned densely across a synthetic genome. Furthermore, this method is extremely expensive, particularly for constructing larger sized synthetic genomes.
The top down genome editing process offers host specific editing systems and is particularly suitable for introducing a few genomic changes quickly and economically. However, this method undergoes multiple editing rounds which increases the risk of off-target editing.
Synthetic Genome Applications
Synthetic genomics has the potential to address various challenges associated with health, environment, and energy. When developed through bottom up strategies, it could lead to the creation of unique cells with distinct biological features such as virus resistance, extremophile tolerances, and metabolic diversity.2,8 These cells could produce valuable bioproducts for industrial applications. Currently, research is ongoing to design synthetic viruses for therapeutics and vaccine development.13
Table: Potential synthetic genome applications14
Areas | Application |
Therapeutics |
|
Agriculture |
|
Environment |
|
Biomaterials |
|
Biofuel |
|
Laboratory Experiments |
|
Bioethics in Synthetic Genomics
Genome synthesis provokes substantial ethical considerations due to the dual-use dilemma, which is the potential for scientific knowledge to be used in both helpful and harmful ways.15 For example, synthetic microbial strains might undergo horizontal gene transfer with exposed natural organisms, which could lead to the unintended expression of synthetic genes outside of a controlled laboratory environment. Therefore, synthetic organisms created only for the laboratory, fermenter, or environmental use are strictly controlled to prevent accidental or unwanted exposures.
One strategy used to identify synthetic organisms is the insertion of 48–143bp nucleic acid signature sequences called watermarks in the designed genome. These watermarks enable easy tracking of synthetic organisms. Considering biosafety, bioengineers develop synthetic genomes in a manner that they cannot share their genetic material with wild type organisms, which potentially reduces risks if accidentally exposed to the environment.16 “Since we can control every nucleotide in the design of the synthetic genome, we can actually design it to be much safer than corresponding wild type organisms,” said Robertson.
Synthetic biologists take bioethics very seriously. “We involve bioethicists to make sure we are doing things responsibly,” said Robertson. “It's part of the process for how we go about designing experiments.” Furthermore, synthetic biologists communicate with researchers across all scientific domains and educate policymakers and journalists to address the concerns regarding synthetic genome applications.
- Zhang H, et al. Investigation of genome biology by synthetic genome engineering. Bioengineering. 2023;10(2):271.
- Fu X, Shen Y. Synthetic genomics: Repurposing biological systems for applications in engineering biology. ACS Synth Biol. 2024;13(5):1394-1399.
- Cello J, et al. Chemical Synthesis of Poliovirus cDNA: Generation of Infectious Virus in the Absence of Natural Template. Science. 2002; 297(5583): 1016-1018
- Mitsunaka S, et al. Synthetic engineering and biological containment of bacteriophages. PNAS. 2022;119(48):e2206739119.
- Gibson DG, et al. Creation of a bacterial cell controlled by a chemically synthesized genome. Science. 2010;329(5987):52-56.
- Sleator RD. JCVI-syn3.0 - A synthetic genome stripped bare!. Bioengineered. 2016;7(2):53-56.
- Fredens J, et al. Total synthesis of Escherichia coli with a recoded genome. Nature. 2019;569(7757):514-518.
- Robertson EW, et al. Sense codon reassignment enables viral resistance and encoded polymer synthesis. Science. 2021; 372:6546
- Dai J, et al. A spotlight on global collaboration in the Sc2.0 yeast consortium. Cell Genom. 2023;3(11):100441.
- Xu X, et al. Trimming the genomic fat: minimising and re-functionalising genomes using synthetic biology. Nat Commun. 2023;14(1):1984.
- LeBlanc N, Charles TC. Bacterial genome reductions: Tools, applications, and challenges. Front Genome Ed. 2022;4:957289.
- Yuan S, et al. Genome-scale top-down strategy to generate viable genome-reduced phages. Nucleic Acids Res. 2022;50(22):13183-13197.
- Bin Umair M, et al. Viruses as tools in gene therapy, vaccine development, and cancer treatment. Arch Virol. 2022;167(6):1387-1404.
- König H, et al. Synthetic genomics and synthetic biology applications between hopes and concerns. Curr Genomics. 2013;14(1):11-24.
- Douglas T, Savulescu J. Synthetic biology and the ethics of knowledge. J Med Ethics. 2010;36(11):687-693.
- Zurcher FJ, et al. Refactored genetic codes enable bidirectional genetic isolation. Science. 2022; 378:6619.