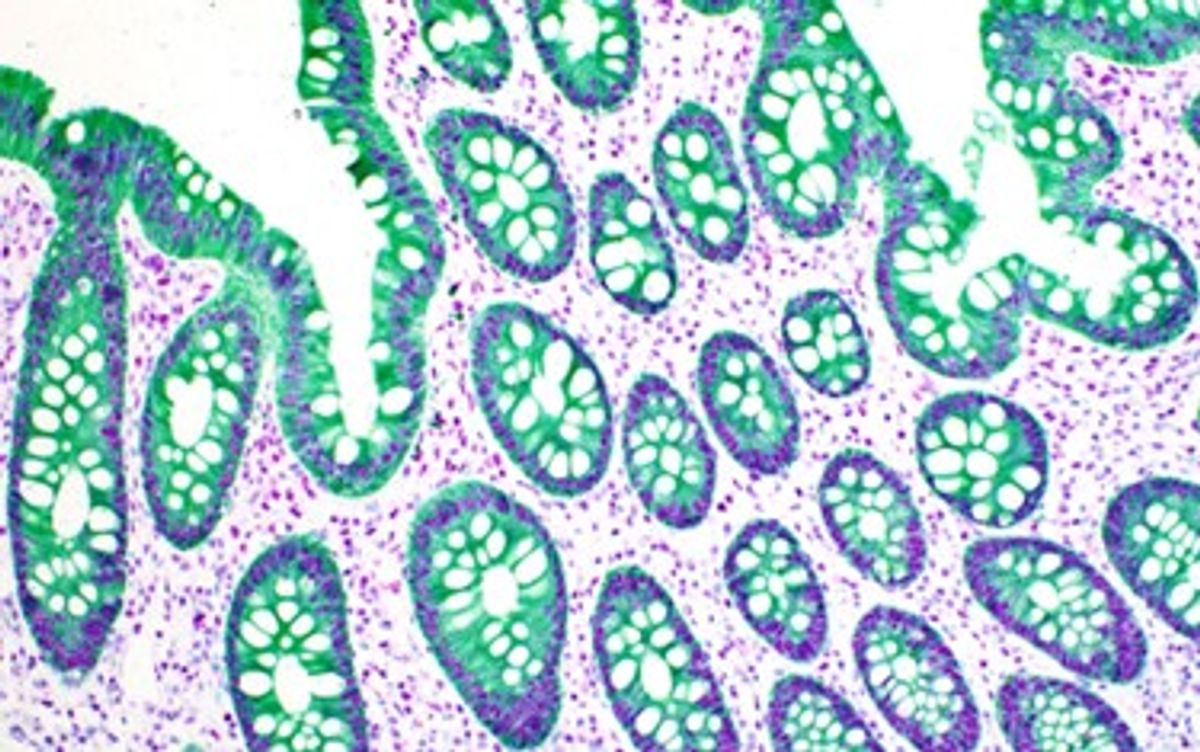
Stay up to date on the latest science with Brush Up Summaries.
Immunohistochemistry (IHC) is a laboratory technique used to detect specific antigens in tissues or cells based on antigen-antibody recognition. A standout feature of IHC compared to other laboratory tests is that it is performed without destroying any histological architecture, allowing researchers to assess the expression pattern of the desired antigen in the context of the microenvironment.1 These data are indispensable as the spatial relationships of cell populations and structures within complex tumor microenvironments have profound implications on the prognosis and potential treatment of disease.2-6
IHC History
The origin of modern IHC methods can be traced back to 1941, when Albert Coons and colleagues first developed a direct staining method using fluorescent-labeled primary antibodies in frozen tissue.7 However, it was not until 1967 that scientists produced enzyme-labeled primary antibodies that allowed for the visualization of target antigens with a standard light microscope.8-11 Since then, a series of technological advancements including utilization of formalin-fixed, paraffin-embedded (FFPE) tissue, methods for antigen retrieval, and advanced detection systems for antibody-antigen visualization has led IHC to become a routine yet essential tool in diagnostic and research laboratories.
Common Applications of IHC
IHC has become an indispensable ancillary technique used in anatomic pathology and basic research laboratories across the globe.12 Pathologists routinely use IHC to aid in the diagnosis and prognosis of disease and as a predictive factor for therapy responses.
For example, tumors have specific markers due to their own unique molecular signatures. As a result, pathologists use IHC to diagnose if a tumor is benign or malignant, identify different types of cancers, such as carcinoma, sarcoma, or melanoma, and pinpoint metastatic tumor origins. IHC can also be used to classify different cancer subtypes, such as lung squamous versus adenocarcinomas. These classifications are important as they better inform the oncologist when determining the most effective therapy for a patient.
Scientists and clinicians also use IHC to determine disease outcomes by providing essential data such as a cancer’s stage and grade and how high-risk or aggressive it is. Additionally, pathologists use IHC to predict whether tumor cells will respond to certain treatments, such as endocrine therapy for breast and prostate cancers.
In basic research, scientists use IHC to study myriad biological processes, including cell proliferation, cell death and repair, tissue and organ development, and gene regulation.
IHC Protocol: General Steps
IHC has traditionally been a manual protocol performed at the bench, and it is still the method of choice in most basic research laboratories. However, most large-scale clinical laboratories have now adopted fully automated protocols using standard autostainers. Regardless of the method used, IHC protocols performed on formalin fixed paraffin embedded (FFPE) tissue are generally similar and can be summarized as follows.
General IHC protocol
1. Deparaffinization and rehydration of tissue
2. Antigen retrieval
3. Blocking
4. Primary antibody incubation
5. Secondary antibody incubation
6. Visualization system
7. Counterstain and Coverslip
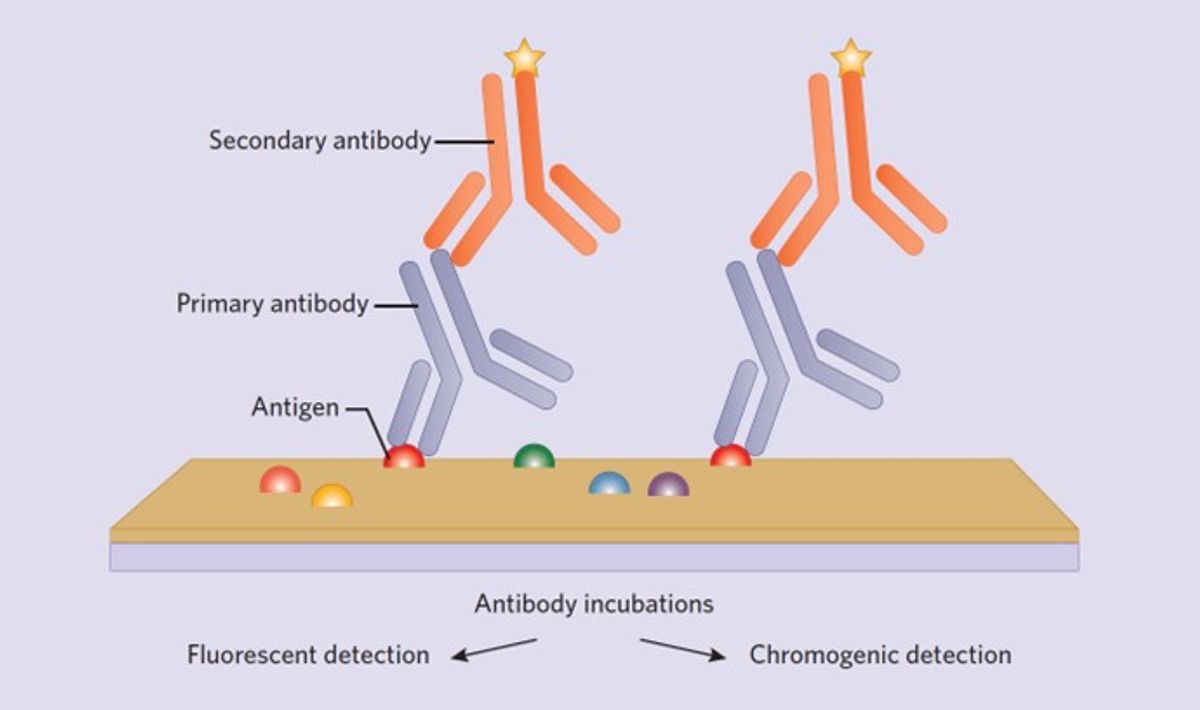
Important staining considerations
While all steps in the IHC protocol affect the quality of the staining outcome, three important factors to consider during initial optimization are primary antibody selection, antigen retrieval, and visualization system selection.
Antibody selection is, arguably, the most important step, as bad antibodies result in bad staining. In general, monoclonal antibodies are recommended over polyclonal antibodies, owing to greater specificity and batch-to-batch consistency. Several online resources are available to help select high quality antibodies.
During tissue fixation, formalin creates methylene crosslinks between the antigens and adjacent unrelated proteins that can physically block antigen-antibody binding. Antigen retrieval is the process of unmasking antigens by breaking these crosslinks, allowing the antibody to bind the antigen of interest. The most common method of antigen retrieval is using heat-based protocols with pH buffers that are either slightly basic or acidic, although proteolytic (enzymatic) options are also available. The best antigen retrieval conditions will likely be different for each antibody and need to be experimentally determined. Traditionally, lower pH retrieval methods work better for manual protocols while higher pH protocols perform better on autostainers.
People working in diagnostic pathological laboratories traditionally favor chromogenic assessment of a single antigen performed on an autostainer. All autostaining platforms use a polymer-based detection system with antibodies conjugated to horseradish peroxidase (HRP) or alkaline phosphatase (AP), which will produce a brown (HRP) or red (AP) chromogen at the site of antibody binding. Polymer detection systems greatly increase IHC sensitivity and significantly reduce protocol time. Additionally, they help meet the high turnaround times required when analyzing patient tissue in clinical settings. IHC protocols in basic research laboratories are generally performed manually with the goal of labeling multiple antigens simultaneously. The current standard for this is using fluorescently labeled secondary antibodies targeted to primary antibodies of interest to be visualized with fluorescent or confocal microscopes.
Interpreting IHC Results
The importance of proper controls and understanding how they are used in IHC cannot be overemphasized.13,14 It is common to encounter both false-positive and false-negative IHC data in clinical and research applications, so scientists must take care when interpreting the results. One tip is to fully understand the expected immunoreactivity (membrane, nuclear, or cytoplasmic) of the antigen of interest and to understand that true-positive stains generally exhibit cell-to-cell heterogeneity.13 However, the best way to interpret IHC results is to always include appropriate positive and negative controls on the same slide as the experimental or patient tissue. Many individuals working in clinical laboratories satisfy this requirement by including a series of tissue cores that are known to be either positive or negative for the antigen of interest. In this context, the results of the patient or experimental tissue can only be trusted when the on-slide controls stain appropriately.
Trouble Shooting Tips for Common IHC Problems
One of the most difficult scenarios to troubleshoot in IHC is addressing a result that is weak, patchy, or lacking signal. Recommended strategies include increasing the primary antibody concentration and incubation time and trying a more aggressive antigen retrieval protocol. Scientists can also employ additional signal amplification methods using kits from major vendors. If all efforts continue to produce sub-optimal results, trying a new primary antibody from a different vendor is recommended.
Another common troubleshooting scenario is decreasing unwanted background staining. Trusted ways to accomplish this are blocking the tissue with either 5-10 percent normal goat serum or casein before one applies the primary antibody. Another trick is to add 0.5M NaCl to the primary antibody diluent. Researchers will need to experimentally determine their incubation times, but such blocking strategies are generally successful in the long run.
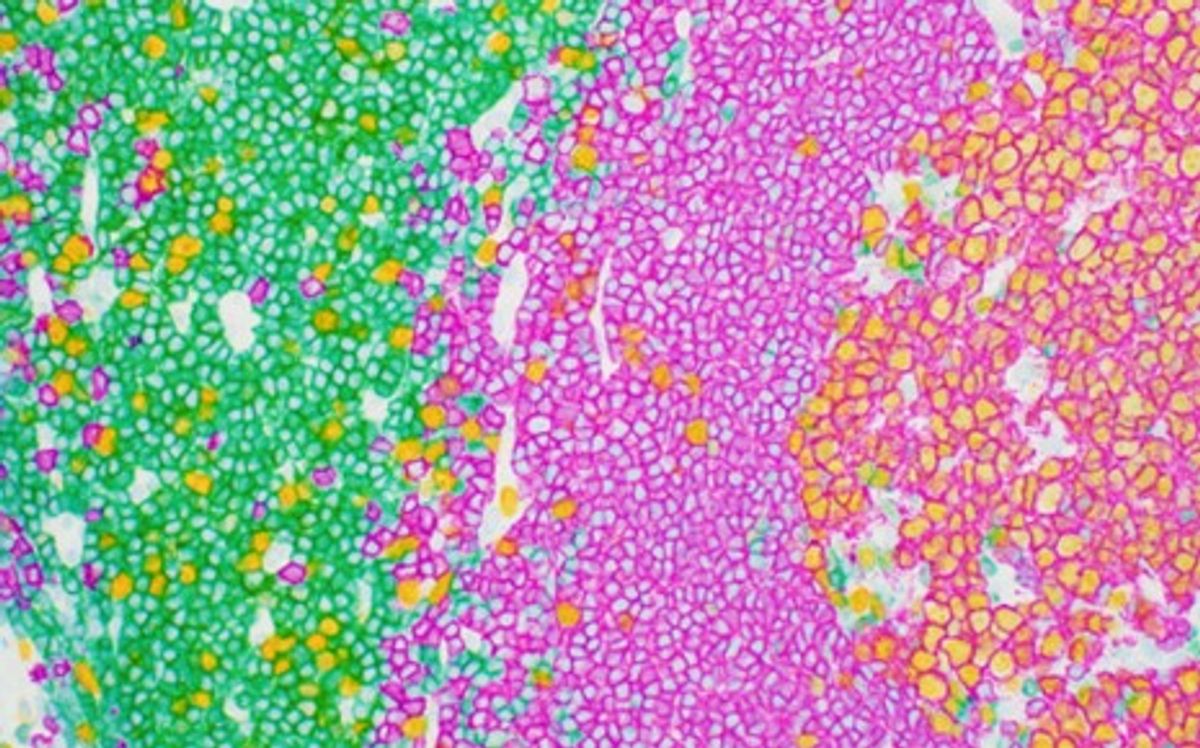
The Future of IHC: Chromogenic Multiplexing of Tumor Microenvironments (TME)
Cancer is not simply a genetic disease, but a complex ecosystem where tumors are involved in a range of interactions with non-cancerous cells in the tumor microenvironment (TME).15 However, tissue-based evaluations of these important regions largely rely upon assessment of single biomarkers using chromogenic IHC. These methods often fail to provide complex quantitative analyses of interactions within the TME.16 For this reason, major biotechnology companies are heavily investing in multiplex IHC approaches using novel colored chromogens to examine complicated landscapes including the TME. New technology has just become available that allows for dual labeling of mRNA and protein simultaneously. Concomitant with the advancement of IHC techniques, sophisticated artificial intelligence is being developed to accurately quantify and digitize the complex data that is produced by these large-scale multiplex approaches. These advancements will undoubtedly become a valuable and innovative tool for anatomical pathologists in the foreseeable future.
- Kim SW, et al. Immunohistochemistry for pathologists: protocols, pitfalls, and tips. J Pathol Transl Med. 2016;50(6):411-418.
- Berry S, et al. Analysis of multispectral imaging with the AstroPath platform informs efficacy of PD-1 blockade. Science. 2021;372(6547).
- Hernandez S, et al. Multiplex immunofluorescence tyramide signal amplification for immune cell profiling of paraffin-embedded tumor tissues. Front Mol Biosci. 2021;8:667067.
- Lu S, et al. Comparison of biomarker modalities for predicting response to PD-1/PD-L1 checkpoint blockade: a systematic review and meta-analysis. JAMA Oncol. 2019;5(8):1195-1204.
- Pages F, et al. International validation of the consensus immunoscore for the classification of colon cancer: a prognostic and accuracy study. Lancet. 2018;391(10135):2128-2139.
- Wharton KA, Jr, et al. Tissue multiplex analyte detection in anatomic pathology - pathways to clinical implementation. Front Mol Biosci. 2021;8:672531.
- Coons AH, et al. Immunological properties of an antibody containing a flourescent group. Exp Biol Med. 1941;47:200-202.
- Avrameas S, Uriel J. Methode de marquage d'antigenes et d'anticorps avec des enzymes et son application en immunodiffusion. C R Acad Hebd Seances Acad Sci D. 1966;262(24):2543-5.
- Marsch AF, et al. A dermatopathologist's guide to troubleshooting immunohistochemistry part 1: methods and pitfalls. Am J Dermatopathol. 2015;37(8):593-603.
- Nakane PK. Simultaneous localization of multiple tissue antigens using the peroxidase-labeled antibody method: a study on pituitary glands of the rat. J Histochem Cytochem. 1968;16(9):557-60.
- Nakane PK, Pierce GB, Jr. Enzyme-labeled antibodies for the light and electron microscopic localization of tissue antigens. J Cell Biol. 1967;33(2):307-18.
- Lin F, Chen Z. Standardization of diagnostic immunohistochemistry: literature review and geisinger experience. Arch Pathol Lab Med. 2014;138(12):1564-77.
- Miller RT. Avoiding pitfalls in diagnostic immunohistochemistry-important technical aspects that every pathologist should know. Semin Diagn Pathol. 2019;36(5):312-335.
- Ward JM, Rehg JE. Rodent immunohistochemistry: pitfalls and troubleshooting. Vet Pathol. 2014;51(1):88-101.
- de Visser KE, Joyce JA. The evolving tumor microenvironment: from cancer initiation to metastatic outgrowth. Cancer Cell. 2023;41(3):374-403.
- Harms PW, et al. Multiplex immunohistochemistry and immunofluorescence: a practical update for pathologists. Mod Pathol. 2023;36(7):100197.
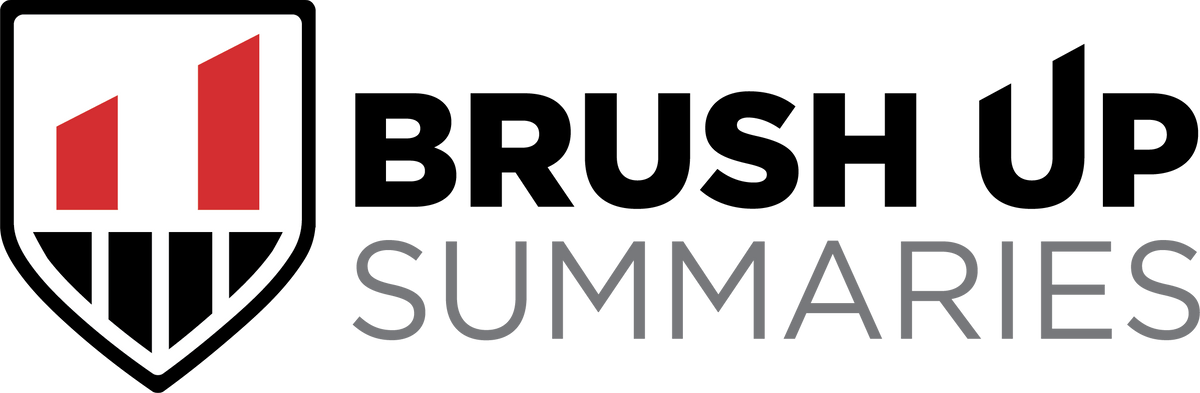