ABOVE: ©ISTOCK.COM, DUSANPETKOVIC
The story of the discovery of penicillin is one of serendipity, promise, and prudence. In 1928, Alexander Fleming returned to his lab at St. Mary’s Hospital in London following a vacation and found something interesting. An agar plate infected with Staphylococcus aureus that he left sitting on a benchtop had a mold growing on it, but the bacteria near the fungus had disappeared. Fleming cultured the mold and observed its remarkable antibacterial properties, later identifying the mold as the genus Penicillium. He published his findings in 1929 (1).
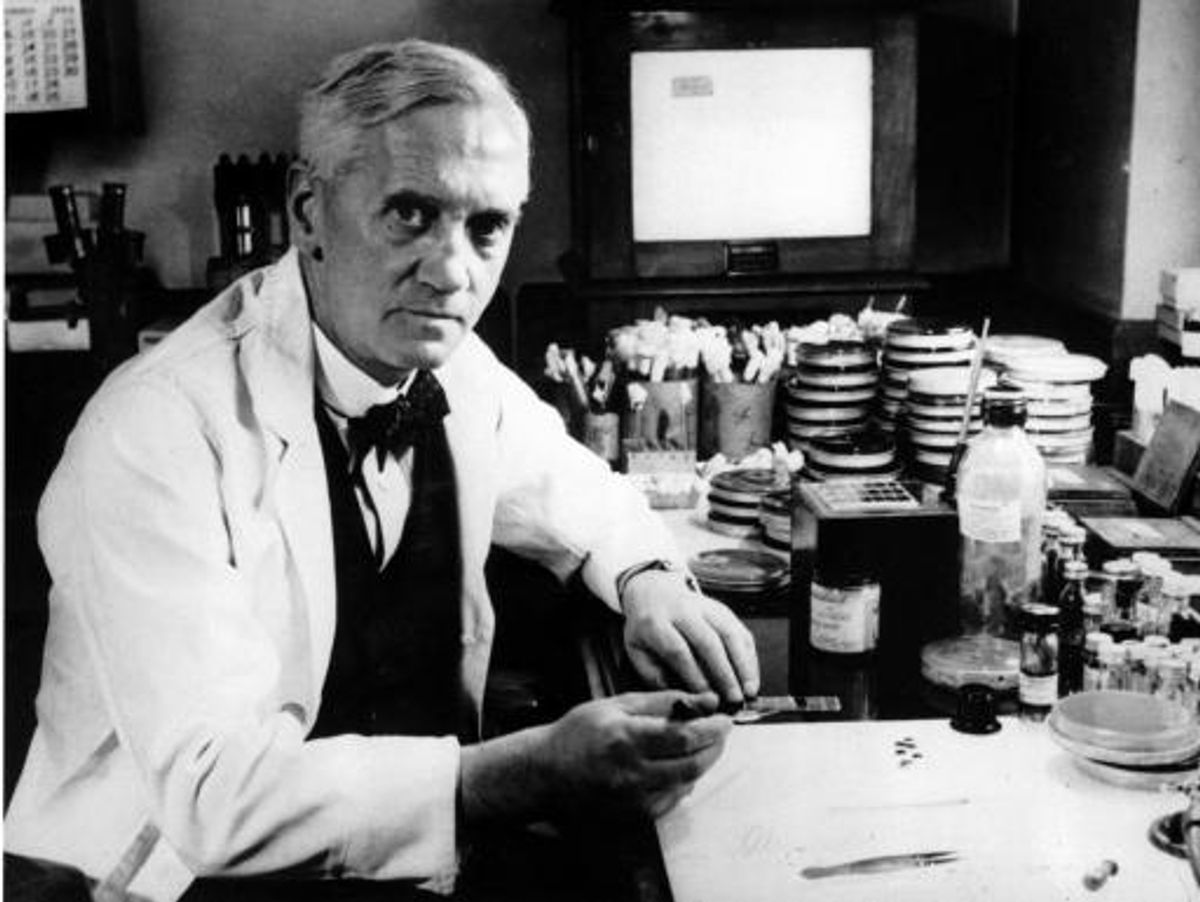
The miracle drug as we know it did not emerge until the early 1940s when University of Oxford chemists Ernst Boris Chain and Howard Florey successfully purified penicillin. Penicillin ushered in the golden age of antibiotics and transformed how we treat disease. Prior to its discovery, life expectancy was low, and an infected scratch could lead to death. After, it saved lives on battlefields and in homes.
Even then, Alexander Fleming, co-recipient of the Nobel Prize for the discovery, warned in his 1945 Nobel Prize speech that misuse of the drug could lead to antibiotic resistance. Indeed, within two years of Fleming’s speech, the first accounts of resistance emerged. Now, antimicrobial resistance (AMR) is one of the greatest threats to modern life. AMR is responsible for nearly 700,000 deaths worldwide each year, and it is projected to kill 10 million per year by 2050.
Antimicrobial resistance predates modern medicine
Bacteria participate in a form of chemical warfare to resist foreign attack. They employ numerous strategies, including bolstering their armor to prevent antibiotics from invading their cell membranes, creating new enzymes to inactivate drugs, and developing specialized pumps to remove antibiotics. “There are so many different ways they can escape. It’s really hard to combat any particular antibiotic resistance mechanism,” said John Dennehy, a virologist at Queens College.
But AMR is not a modern phenomenon. It is part of a long-standing evolutionary process where bacteria constantly adapt to survive in their environments. In fact, a group of scientists from McMaster University unearthed an antibiotic resistance gene in 30,000-year-old permafrost samples (2). Although humans did not create AMR, we have amplified these natural resistance mechanisms by creating numerous selective pressures for resistance, largely through the misuse of antibiotics in medicine and agriculture.
Scientists warn that we need a novel approach to contain the rising rates of AMR, but there isn’t a magic bullet. A combination of factors, including novel treatments, increased surveillance, and antimicrobial stewardship must all be part of the solution.
Treatments
Unclogging the pipeline
The latest World Health Organization (WHO) annual Antibacterial Pipeline Report details how regulatory agencies have only approved a few antibiotics over the last few years, with 82% of these compounds in the same family as existing antibiotics with proven drug resistance. “Ultimately, efforts to find new drugs keep leading us to the same place,” said Fabrizio Spagnolo, a postdoctoral researcher working with Dennehy.
There is a dire need for novel antibiotics, but the high development costs and failure rates pose substantial risks for pharmaceutical companies.
Turning a phage in the antibiotic playbook
In 2012, ophthalmologist Ali Khodadoust underwent a coronary artery bypass, following which his situation rapidly turned south. The surgeons at Yale New Haven Hospital returned to the operating room to find a green film covering his aorta; they later identified this as a biofilm of superbug Pseudomonas aeruginosa.
Doctors started Khodadoust on an aggressive antibiotic regimen, but nothing helped. After Khodadoust battled the infection for years with little hope, Benjamin Chan, a scientist at Yale University, proposed an experimental treatment: phage therapy (4).
Bacteriophages, also known as phages, are viruses that infect bacteria. Following their discovery in the early 1900s, bacteriophages gained enthusiasts around the world who were intrigued by their antimicrobial potential (5). The discovery of penicillin cut short these efforts in much of the western world, but research continued in the Soviet Union. Decades later, phage therapy is now experiencing a renaissance in the West.
Bacteriophages have two modes: kill or coexist. Much of the allure of bacteriophages is their ability to induce pathogen-specific cell death. There are a variety of bacteriophages, but like a lock and key, a specific bacteriophage can only bind to bacteria expressing a specific receptor. This means that bacteriophages only attack bacteria with their receptor match, in contrast to traditional broad-spectrum antibiotics.
Once they bind to receptors on bacterial cells and insert their DNA, bacteriophages continue to replicate inside the cell. “At the end of the replication process, the phages lyse open the cell, causing it to burst open, which is visually amazing,” said Dennehy. “It’s like a balloon popping because the cell is under pressure.”
While antibiotic concentrations gradually decrease over time, bacteriophages exponentially increase, reinforcing their efforts.
The specificity of bacteriophages is a double-edge sword. More targeted attacks mean fewer side effects, but finding a bacteriophage that holds the key to a particular pathogen is difficult.
Going on a phage hunt
Bacteriophage live everywhere bacteria live: soil, lakes, sewage, oceans, and compost. In his efforts to heal Khodadoust, Chan embarked on a “phage hunt” around Connecticut, collecting samples and testing them against P. aeruginosa. Finally in a pond near New Haven, Chan found the bacteriophage he hunted for so long. Named OMKO1, this bacteriophage worked by blocking the pumps P. aeruginosa used to remove antibiotics (6).
Four years after his infection began, Khodadoust returned to the operating room to receive this experimental treatment. The surgeons applied a syringe full of OMKO1 bacteriophage and antibiotics directly to the infected area, and Khodadoust began his journey to recovery.
Chan eventually chanced upon the OMKO1 bacteriophage, but the search for useful phages is open-ended. Given that phage therapy is considered a last resort treatment, time is of the essence.
Compounding this, more than a receptor match is required for bacteriophage to successfully infect. Once a bacteriophage gains access to the cell, it needs to be compatible with the host’s replication machinery, and specialized enzymes can attack the phage. “There are all sorts of things that could go wrong once that phage gets inside,” said Dennehy.
Waging a war from within
Instead of searching high and low for a perfect phage match, Dennehy and Spagnolo now research the therapeutic potential of prophages, the other class of bacteriophage that coexist peacefully with bacteria.
Prophages can share beneficial genes to contribute to bacterial fitness and even protect them against bacteriophage attacks. Dennehy and Spagnolo are interested in whether they can somehow trick these prophages to enter their lytic cycle, killing their hosts in the process. The researchers describe this process as a “smoke them out and kill in place” approach.
Dennehy’s team recently received an NIH bacteriophage research grant to further explore the potential of leveraging prophages to kill bacteria like P. aeruginosa, whichlike to live in difficult-to-treat biofilms.
“We’re really at the very start of this story, said Spagnolo. “If you compare it to the antibiotics story, we are not even up to Fleming yet.”
Surveillance
Novel treatments alone will not stave off rising rates of AMR infections. One thing the COVID-19 pandemic has revealed is the need for global surveillance systems to track emerging infections.
In 2015, the WHO launched the Global Antimicrobial Resistance Surveillance System (GLASS) to encourage countries worldwide to track AMR and antimicrobial use with the hope that this information will help direct resources and inform policy.
“You can’t manage what you can’t measure,” said Thomas van Boeckel, a spatial epidemiologist at ETH Zurich. “To set targets for more stringent regulation of antibiotic use, which is to measure how fast antimicrobial resistance is rising, you need to have a monitoring system of some kind.”
For the last decade, van Boeckel has worked on issues related to automating epidemiological data collection and mapping the geographic distribution of antimicrobial use and resistance genes. However, his team’s focus is not on humans, but pigs, chickens, cattle, and fish.
More than 70% of the antimicrobials sold worldwide are used in animal production as a means to fatten up livestock and prevent infection due to poor hygiene (7). As the global demand for meat continues to rise, so also will antimicrobial misuse. Of particular concern is the growing evidence linking antimicrobial overuse in livestock with AMR infections, not only in livestock, but also in humans (8, 9).
Despite the levels of antimicrobial use, there is no GLASS equivalent for tracking AMR in livestock. With no way of identifying hotspots for antimicrobial use or regions with emerging resistance, controlling the situation is difficult. To start laying the building blocks for such a system, van Boeckel analyzed prevalence surveys of AMR in animals from around the world, and used geospatial models to create global maps of AMR (10). These findings provide a starting point for tracking AMR levels in animals and identifying potential regions for targeted interventions.
Education
The plight of the boiling frog
The last 18 months of the COVID-19 pandemic have uncovered the lack of preparedness for a public health crisis, the grave inequalities that exist in access to treatment, and the need for stronger scientific communication and education. While there are many parallels between COVID-19 and the looming AMR pandemic, there is a difference in public awareness. Much like a frog placed into boiling water, society mounted a rapid escape response to the current pandemic. However, antimicrobial resistance has so far lacked such a response, but the water is heating up.
“There has been a slow reaction, and I think it’s largely because we can’t see it,” said Roger Harrison, an epidemiologist at the University of Manchester.
Much of Harrison’s work shines a light on the issues surrounding AMR using evidence-based practice, education, and public engagement. For example, Harrison and colleagues recently launched an open-source mini-course to foster a general understanding of AMR and its impact on society. The course includes modules focused on tackling common misconceptions surrounding AMR, such as when it is and is not appropriate to use antibiotics, and explains how simple hygiene can save lives.
“We don’t need to have an extensive knowledge about microbiology or pharmacology; we just need to understand the very basics,” said Harrison.
Crowdsourcing action in an increasingly connected world
A trip to a local Manchester primary school to discuss antimicrobial resistance inspired Harrison to further pursue public health education. He was surprised by how much the children knew, and was encouraged by the challenging questions they posed to him.
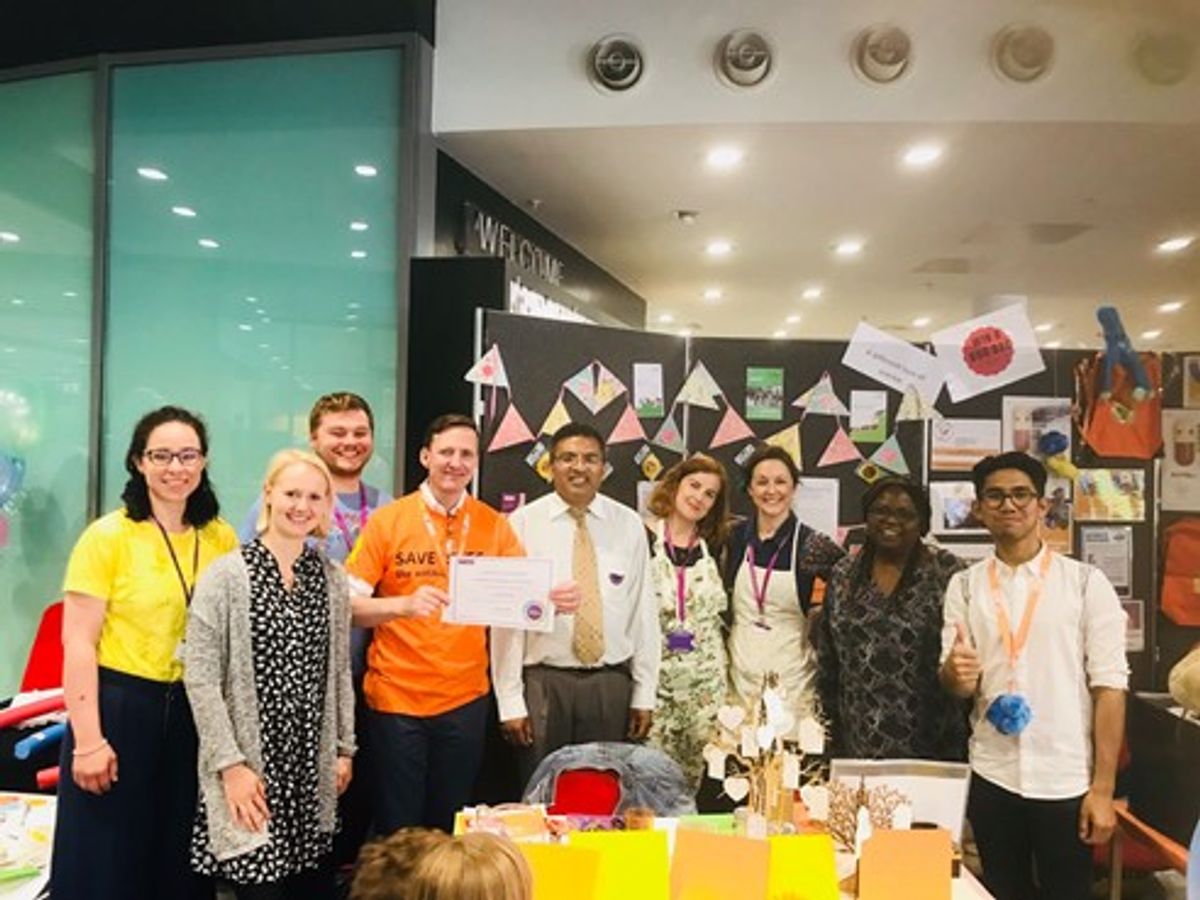
As a result, Harrison applied for and received funding to pilot a workshop that trains university students on communicating science to the public. Harrison modeled the program off of crowdsourcing principles. Much like a single phage replicating exponentially in the bacterial war, a single student can educate five people about AMR, and each of these individuals can educate another five people, and so on.
Further opportunities for public engagement came about through conversations Harrison had on Twitter with medical students in Uganda and Tanzania who were interested in developing education campaigns tailored to their communities. The initiatives that grew from this use everything from local radio campaigns, tailored workshops, and school clubs to empower individuals to be agents of change.
Reducing the risks of AMR will require a coordinated effort by organizations and individuals in every sector of society. While doubling down on research and policy is necessary, it is also important to consider the impact of infrastructure, or a lack thereof, on the AMR crisis. Harrison said that a lot of the basics have been lost in the pursuit of science and science teaching. The most life-saving measures are often fundamental preventative measures, including washing hands and foods adequately. However, behavioral change can only occur when resources like clean water are available.
Recognizing the interconnection between animal, human, and environmental health forms the basis of the One Health approach to public health championed by world leaders in disease prevention. Investing in research and surveillance systems, advocating for evidence-based policy, and educating farmers, doctors, and the public on antimicrobial use are all needed to avert a future pandemic.
“What happens in Uganda will influence what happens in Manchester, and what happens in Manchester will influence what happens in Uganda,” said Harrison. “More and more, we have become globally connected.”
- Fleming A. On the Antibacterial action of cultures of a Penicillium, with special reference to their use in the isolation of B. influenzae. Br J Exp Pathol 60, 3-13 (1979).
- D’Costa, V., King, C., Kalan, L. et al. Antibiotic resistance is ancient. Nature 477, 457-461 (2011).
- Mahase E. UK launches subscription style model for antibiotics to encourage new development. BMJ 369, m2468 (2020).
- Chan, B., Turner, P.E., Kim, S., et al. Phage treatment of an aortic graft infected with Pseudomonas aeruginosa. Evol Med Public Health 1, 60-66 (2018).
- Stone, R. Stalin’s forgotten cure. Science 298, 728-731 (2002).
- Chan, B., Sistrom, M, Wertz, J.E., et al. Phage selection restores antibiotic sensitivity in MDR Pseudomonas aeruginosa. Sci Rep 6, 26717 (2016).
- Van Boeckel, T., Glennon, E.E., Chen, D., et al. Reducing antimicrobial use in food animals. Science 357, 1350-1352 (2017).
- Liu, Y., Wang, Y., Walsh, T.R., et al. Emergence of plasmid-mediated colistin resistance mechanism MCR-1 in animals and human beings in China: a microbiological and molecular biological study. Lancet Infect Dis 16, 161-168.
- Liu, C.M., Stegger, M., Aziz, M., et al. Escherichia coli ST131-H22 foodborne uropathogen. mBio 9, e00470-18 (2018).
- Van Boeckel, T., Pires, J., Silvester, R., et al. Global trends in antimicrobial resistance in animals in low- and middle-income countries. Science 365, eaaw1944 (2019).
This story was originally published on Drug Discovery News, the leading news magazine for scientists in pharma and biotech.