Building Living Bridges with Anthrobots
Researchers used adult human cells to craft biological robots capable of movement and more.
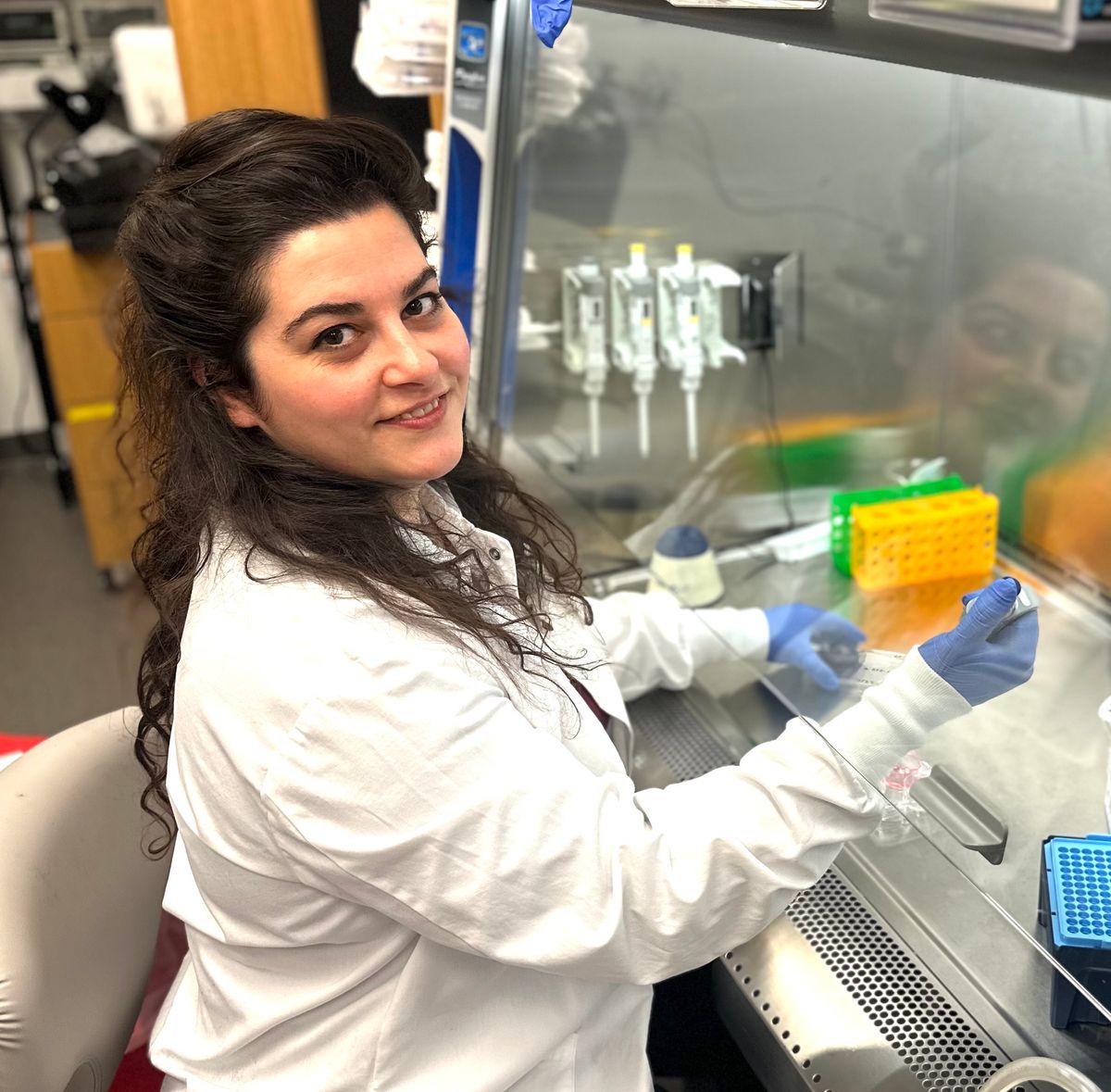
Growing up in Turkey, synthetic biologist Gizem Gumuskaya was enamored with architecture and how buildings emerged from the bustling chaos of the city. She found that designed environments mirrored biology, as nature, the ultimate architect, constructs complex structures from a single cell.
This led her to seek graduate research that harnessed biology as a working medium in Michael Levin’s lab at Tufts University. Intrigued by Levin and his colleagues’ previous work, which combined artificial intelligence designs and embryonic frog cells to create living biological robots that could swim and self-replicate, Gumuskaya was eager to test whether this would function in a non-froggy model.1 “I wanted to build something that evolution didn’t already design for us,” she said.
She combined her engineering mindset and the self-organization of biology to create mammalian-derived living robots, anthrobots, from adult human tracheal cells.2 The adult cells displayed morphologic plasticity and self-assembled into ciliated spheres that enabled them to wiggle and swim through their environments. Not only could they move, but anthrobots spontaneously fused to form larger structures called superbots.
When Gumuskaya explored the anthrobots’ influence on other cells, the results surprised her. When they studied images of these cultures, they saw that these superbots formed a bridge-like structure that connected to a layer of damaged human neurons. This structure resembled an ant bridge, a concept from Gumuskaya’s architecture studies. Like how ant colonies function as a superorganism, the superbots showed enhanced capabilities of teamwork, collectively aiding in the healing of damaged neurons. However, the exact mechanism remains unclear, Gumuskaya noted.
Anthrobots, an extension of their amphibious predecessor, hold promise for biological applications. “Anthrobots are just one example of what we can accomplish by thinking about nature as a design medium. I hope the scientific community will look at cells through this lens and imagine how to create other machines,” remarked Gumuskaya.
Correction: August 19, 2024. The story has been updated to accurately reflect Gumuskaya's affiliations and projects.
- Kriegman S, et al. Proc Natl Acad Sci USA. 2020;117(4):1853-1859.
- Gumuskaya G, et al. Adv Sci (Weinh). 2024;11(4):e2303575.