ABOVE: After years of research, brain organoids now come close to mimicking endogenous brain cells. © iStock, StockSnap
As a developmental neurobiologist at Harvard University, Paola Arlotta spends most of her time thinking about how the brain develops, how it functions, and what goes wrong in the context of neurological disease. Using human brain organoids as a model for brain development and disease research has been a game changer, providing Arlotta a novel view into brain pathologies that form in utero.
Why are human-centric models important for understanding the brain?
The human cerebral cortex has evolved to be quite different from that of other animals such as rodents, which are typically used by researchers to understand normal brain development and pathology. A lot of drugs that should target the human brain and treat human diseases fail once they get to clinical trials. This is partially because they were developed using animal models, and the mouse brain is not the human brain. The human brain has specializations that make it unique, including the way the cells and circuits respond to therapeutics or perturbations.
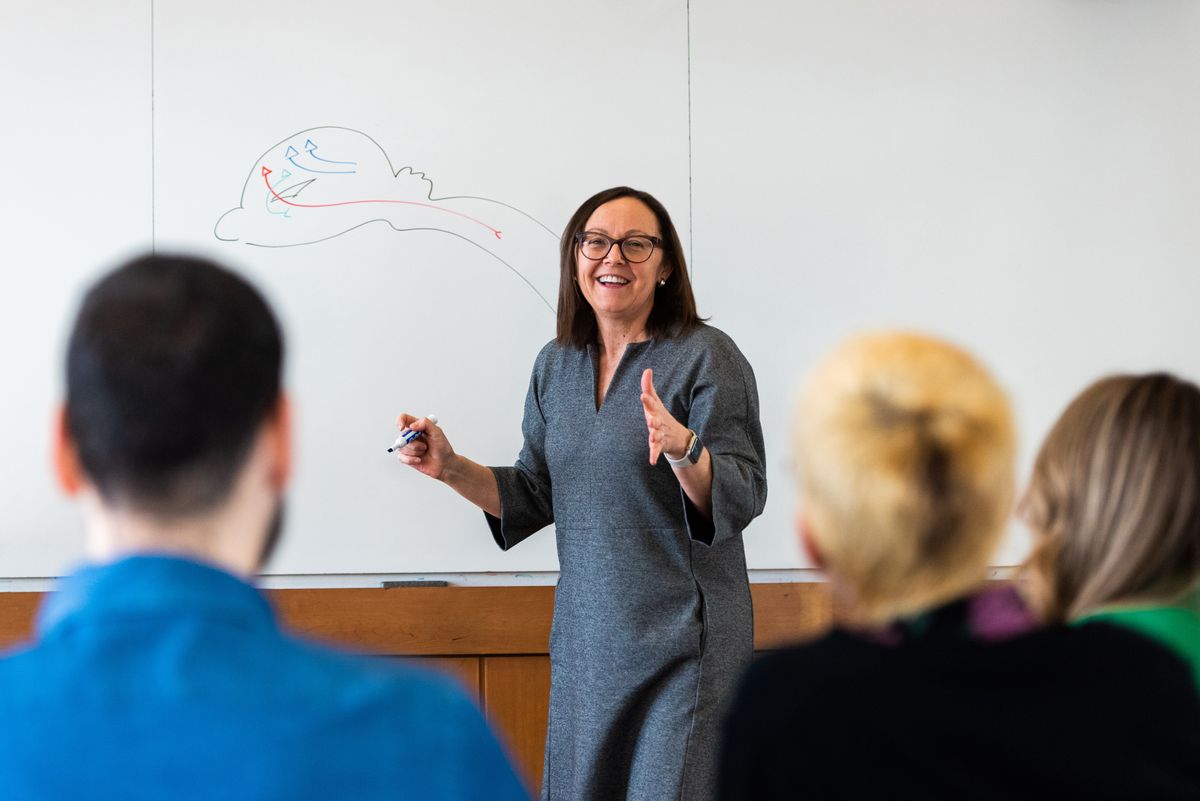
Genetic studies of complex neuropsychiatric and neurodevelopmental diseases have shown that the majority of patients with these diseases have mutations in more than one gene. There are small differences in the genome that increase the probability of disease development. These are diseases of the human genome, so researchers cannot model these in cells that carry the mouse genome. We need the human brain, but rare samples of postmortem brain or small biopsy samples collected during surgery are not experimental models because they cannot grow more brain. These samples only show a snapshot in time for the specific brain region they came from in individual patients.
How has brain research evolved in recent years?
The human brain organoid field started a little over 10 years ago, when researchers including Yoshiki Sasasi at Kyoto University Graduate School of Medicine and Juergen Knoblich and Madeline Lancaster at the Institute of Molecular Biotechnology of the Austrian Academy of Science produced the first examples of brain-like tissue from stem cells using signals in 3D cell culture that drive neural development.1,2 From there, many groups, including ours, have figured out how to extend that process from simple tissue to cultures that can grow over long periods of time. This is what it takes to make a complex structure with different cell types, networks, and synapses that fire properly. It is like watching a movie of development from a stem cell all the way to a brain replica in the form of an organoid. In parallel with this incredible advance came the single cell genomics revolution. Now scientists can sequence and understand the molecular fingerprint of every cell in any tissue. These developments gave us the opportunity to study tissues that we did not understand very well.
How do brain organoids compare to real brains?
Brain development is like a symphony, where many instruments have to play together in harmony.
–Paola Arlotta, Harvard University
Every time an embryo is made, it goes through the same developmental steps to create similar features. The cellular composition of my brain will be similar to yours. This was not the case for organoids at first; we could not predict how they would turn out. For human organoids to be experimental models for brain development, we had to make sure that the process to form them in any laboratory was reproducible, and that every brain organoid went through the same developmental process to produce something that was identical in cellular composition.
Now we have organoid models that we can trust that are close to the endogenous brain. We had to figure out culture conditions that would control cellular self-organization, differentiation, and all the steps in between. At single cell resolution, we compared cell atlas data from developing fetal human brain samples to our organoids and found that they were similar.3
You recently used brain organoids to study the genetic risk for autism spectrum disorder. How did that project come about?
This was one of the most difficult pieces of work that we ever did in terms of technical and analytical difficulty. A team of postdoctoral researchers in my laboratory decided to work together to discover something unknown about autism spectrum disorder genetic risk.4 We started with three rare single mutations in different genes that associate with autism risk, but nobody knew what processes in the brain they affect. We grew organoids with the same genetic background from stem cells with or without the different mutations. Then we used single cell RNA-seq and assay for transposase-accessible chromatin with sequencing (ATAC-Seq) to look at gene expression in specific cell types and the timing of cell production.
What did you learn about brain development and autism spectrum disorder from this model?
A few surprising things emerged that left us puzzled. There were some cell types or neuron populations within the organoids that were consistently affected by all three mutations. Also, it seemed that the timing of when the neurons were made was off. There was a period of time early on during organoid development where the mutants made the cells either too quickly or too slowly.
Brain development is like a symphony, where many instruments have to play together in harmony. If there is one instrument playing faster or slower than the other instruments, the music they produce is different. During development, if there is a cell that forms too soon or too late, the brain will function differently, and the circuits in the neural networks may not function properly. Without organoids, we never could have caught this insight into early developmental events that happen in the second trimester of pregnancy or even earlier.
Many neurodevelopmental diseases likely have problems with their neural networks. Understanding how a circuit works and what circuit properties may be off in disease may offer an opportunity for developing drugs to correct those differences. Human organoids are now becoming cellular platforms on which we can screen for drugs given a defect associated with a disease.
Where is brain organoid research heading in the future?
Organoids now make it possible to investigate many brains from many different people. Not only is my brain different from that of a mouse, but my brain is also different from your brain. So, when I grow an organoid from an individual’s stem cell, I only have the genome of that individual. The reality is that we are genetically distinct and we respond to drugs in different ways.
We recently produced a human organoid that we called a Chimeroid, which we generated from different people’s stem cells.5 By treating Chimeroids with drugs or creating perturbations, we can compare the responses of certain cells from one person’s brain to another’s. This type of biological data can feed into generative models to predict how an individual’s cells will react to a drug, for example, even before going into a clinical trial. In a world of artificial intelligence and modeling, these data can improve our understanding of diseases and drug development. That’s what is exciting about the future, and it all was born of this ability to coach a stem cell to become brain tissue and then improving upon that system over the years.
This interview has been condensed and edited for clarity.
- Kadoshima T, et al. Self-organization of axial polarity, inside-out layer pattern, and species-specific progenitor dynamics in human ES cell–derived neocortex. PNAS. 2013;110(50):20284-20289.
- Lancaster MA, et al. Cerebral organoids model human brain development and microcephaly. Nature. 2013;501(7467):373-379.
- Uzquiano A, et al. Proper acquisition of cell class identity in organoids allows definition of fate specification programs of the human cerebral cortex. Cell. 2022;185(20):3770-3788.e27.
- Pigoni M, et al. Cell-type specific defects in PTEN-mutant cortical organoids converge on abnormal circuit activity. Hum Mol Genet. 2023;32(18):2773-2786.
- Bolaños NA, et al. Multi-donor human cortical Chimeroids reveal individual susceptibility to neurotoxic triggers. BioRxiv Published online October 6, 2023:2023.10.05.558331.