ABOVE: Therapeutic vaccines against cancer could one day join patients' tumor treatment options. © istock.com, Kuzmik_A
Cancer treatments have continuously improved with increasing knowledge about the tumor and the immune system. Starting with surgery and radiation as effective interventions, followed by targeting rapidly diving cells with chemotherapy, researchers gradually expanded the range of cancer treatment options.
More recently, they turned to immunotherapy to target tumors more precisely. These approaches include monoclonal antibodies against tumor antigens, checkpoint inhibitors that block tumors from accessing suppressive proteins on immune cells, and chimeric antigen receptor (CAR) T cells engineered to recognize specific tumor antigens. Each of these approaches uniquely targets tumors to stop their growth or even kill the cancerous cells. However, these immunotherapeutic modalities involve targeting a singular antigen on tumors, and no therapy to date provides long-lasting immunity against possible future tumors, opening the possibility of tumor escape and re-emergence once therapy stops. Vaccines against cancer offer a potential solution to both problems.
Vaccinating against yourself
Researchers have explored the possibility of using vaccination as a therapy against cancer since the 1990s.1 However, despite their appeal, turning the immune system into a therapy against cancer has proven to be challenging.
Cancer vaccines can either be preventative or therapeutic. Currently approved preventative cancer vaccines include the hepatitis B vaccine and human papillomavirus vaccine, both of which generate immunity against a specific virus that can cause cancer after infection. However, developing a preventative vaccine against spontaneous tumors is a much taller task.
“The day before a cancer cell develops, it is a normal cell,” said Christopher Schliehe, an immunologist at Erasmus University Medical Center who studies mechanisms of antigen presentation through major histocompatibility complex class I (MHC-I). “You cannot vaccinate against that because it’s yourself.”
Unlike viruses and bacteria, which have proteins and sugars that scientists can target for a vaccine, it is not currently possible to predict what mutation will occur in a cell to render a tumorigenic protein to formulate a vaccine against. Instead, researchers are interested in a model of vaccination that is used therapeutically.
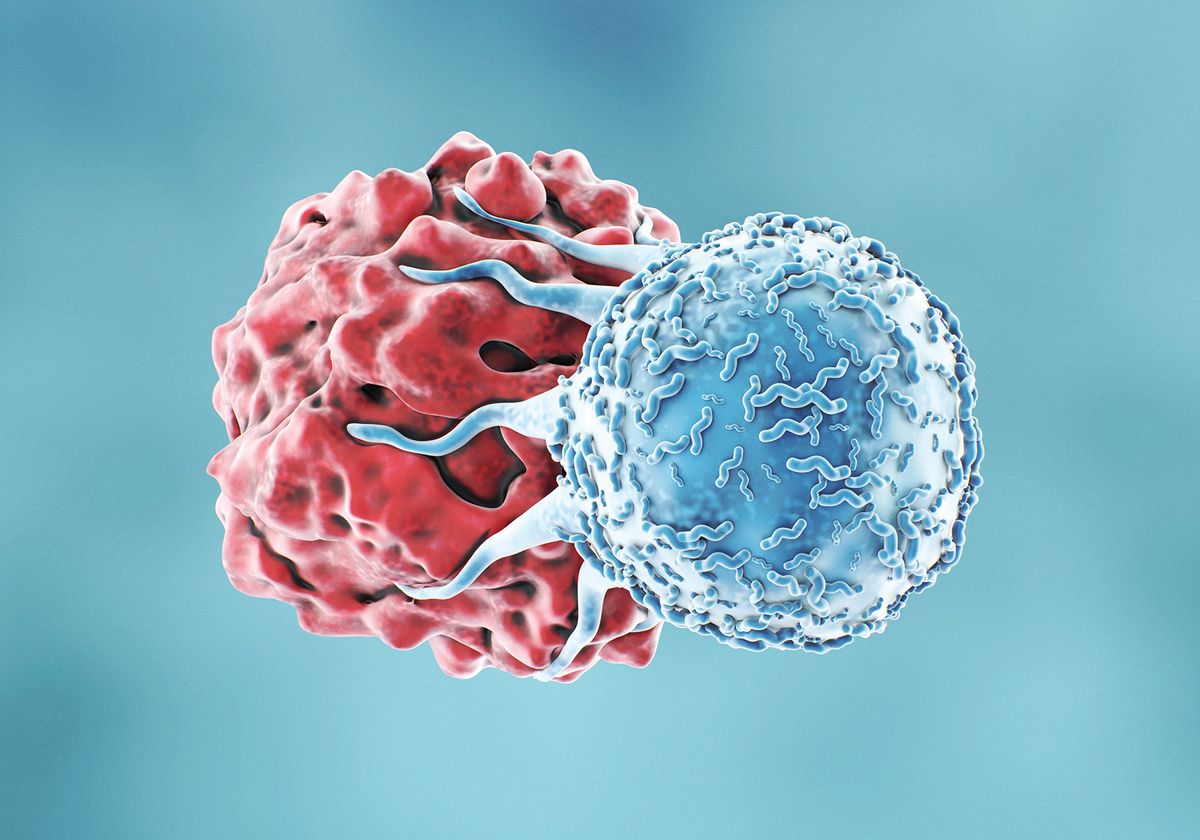
A therapeutic cancer vaccine introduces one or more tumor antigens to the immune system and prompts these cells to activate against the antigens, in turn, targeting and destroying the tumor. Tumor antigens come in one of two categories: tumor associated antigens (TAA) and tumor specific antigens (TSA).2 TAA are found on normal cells but at much lower densities than on cancerous cells. They can be more general targets across different patients’ tumors, making them ideal for a generalized vaccine goal, but because they are a self-protein, there are few T cells available to recognize these antigens. In contrast, TSA arise from mutations that alter one or more amino acids in a protein. This increases the likelihood of T cells recognizing these antigens but makes them more specific to an individual’s tumor.
Studies in the 1970s that used inactivated tumor cells combined with bacterial components, often the live vaccine bacillus Calmette Guérin, yielded conflicting results.3,4 However, this concept truly began taking form with the discoveries of dendritic cells’ functions in antigen presentation and stimulation of T cells, coupled with the identification of mutant antigens in tumors.5-9 Researchers incubated dendritic cells isolated from patients with either whole tumors or specific tumor peptides and then reintroduced the cells into patients.1, 10-12 These antigen presenting cells activated T cells against the tumor targets.
“It’s a type of immune therapy that will persist for a long time,” said Eli Gilboa, a cancer immunologist at the University of Miami who studied RNA-pulsed dendritic cells as vaccine candidates.13,14 Since this therapy introduces functional antigen-pulsed dendritic cells into patients, the activated T cells also produce memory T cells analogously to immune responses against infections or preventative vaccines.15,16 This could provide long-term protection against tumor re-emergence.
However, similar to preventative vaccines against an infectious disease, the antigen chosen for a cancer vaccine is important. “One of the considerations is targeting something that the tumor needs to stay a tumor,” said Erika Crosby, a cancer immunologist at Duke University who studies the tumor microenvironment for developing therapeutic approaches. Whether researchers target a TAA or TSA, some of these mutated or upregulated proteins are more responsible for the cell’s abnormal growth and survival than others and are referred to as “drivers.” While a tumor may evade immune recognition by ceasing expression of certain antigens, it cannot easily stop producing its drivers, so researchers target these proteins that are more likely to be available for the immune system to recognize.17
Additionally, because therapeutic vaccines consist of antigen presenting cells that activate T cells, this becomes a dynamic therapy that can adapt to the tumor. As tumors die, they expose new antigens that dendritic cells can use to activate new T cells.18 This further reduces the likelihood of tumors evading this type of therapy by altering their antigens. “An effective anticancer vaccine is not only going to allow us to put pressure on maybe a driver antigen or multiple driver antigens in tumors, but also allow us to get this epitope spreading,” Crosby explained. “That is going to help us keep fighting the tumor as it evolves.”
Vaccinating Against TumorsCancer vaccines offer the opportunity to use the patient’s own immune system in the fight against tumors. ![]() modified from © ISTOCK.COM, ttsz, Rungnaree Jaitham, Shonyjade, PsychoBeard; designed by ashleigh campsall 1. Antigen preparation: Scientists can produce cancer vaccines against tumors by using the patient’s tumor as a source of antigen or by synthesizing peptides from tumor antigens. 2. Antigen delivery: Researchers use different vaccine platforms to deliver the antigens: patient-derived dendritic cells, adeno-associated viral vectors, and lipid nanoparticles. 3. Antigen presentation: Once administered, these antigens eventually find their way to patient dendritic cells, or are immediately expressed on dendritic cells if they are used as the vehicle. The dendritic cells travel to lymph nodes to activate antigen-specific T cells. 4. Anti-tumor immunity: Once activated, these tumor-antigen-specific T cells will multiply (a) and travel to the tumor, where they help target the tumor cells for destruction (b). Some activated T cells will become memory cells (c, shown in purple); these will reside in the lymph node or nearby tissue to protect the body against future recurrences of the same type of cancer. |
The tumor challenge
Despite the promise and potential advantages of therapeutic cancer vaccines, only one, Sipuleucel-T, a dendritic cell-based vaccine, has received Food and Drug Administration approval in almost thirty years of investigation.19 The dendritic cell-based platform requires the ability to isolate, prepare, and then administer adequate numbers of antigen-pulsed cells for each individual patient, which is both labor-intensive and costly, making them less attractive to pharmaceutical companies.20,21 Although improved generation methods and the ability to outsource these efforts alleviated some of the strain, these therapies are still not as widely applicable as other immunotherapies that can be used in more patients and have shown more progress in clinical trials.22,23
While the previous decade saw the landmark approval with Sipuleucel-T, it still does not cure cancer and only extends survival by four months on average. In many other clinical trials, researchers experienced similar setbacks, where despite demonstrating safety and generating responsive cells, these formulations failed to significantly improve cancer outcomes.24
“To elicit an effective immune response against cancer is particularly challenging because the cancer is expecting an immune response,” Gilboa said. These overactive cells must fly under the radar of the immune system to survive, so many of these cells have developed mechanisms to evade immune defenses. “The tumor microenvironment is so suppressive that you could pour T cells on top of it, and they’re not going to be able to do anything,” Crosby said.
Even if tumor-targeting T cells access the individual tumor cells, they often struggle to engage with their targets because many cancer cells reduce the expression of key immune molecules, such as MHC-I, to evade immune detection. “You can have the best T cell response, and you can have the best vaccine or the best transgenic T cell therapy, but if the tumor itself is not presenting the antigen on MHC class one, then we have a big problem, because then the T cells cannot see the targets,” said Schliehe.
Old problems, new tricks
For vaccine-generated immune cells to effectively attack and eliminate tumor cells, they must be able to penetrate the suppressive tumor environment and access their targets. This is easier to accomplish if treatments can get an early start before the tumor has a chance to establish its defenses.
“You have to think about all the things that the cancer has done to the immune response to favor its survival, and that’s a tough mountain to overcome,” said Brian Czerniecki, a physician scientist at Moffitt Cancer Center. “For cancer vaccines, the earlier they are used, the more effective they will be.”
Czerniecki treats patients with breast cancer, and like many others, he targets human epidermal growth factor 2 (HER2), a driver protein that is shared across many patient tumors, using dendritic cell-based vaccines. However, in his previous clinical trials, he recruited patients who were in the early stages of cancer and administered the vaccine to the patients’ lymph nodes, as opposed to intravenously or intramuscularly, prior to them starting chemotherapy. He found that this cancer vaccination approach drastically shrunk tumor sizes.25 Czerniecki also found that intratumoral vaccination in mice effectively reduced tumor sizes, offering another promising option for patients in the future.26 “You can actually make this stuff disappear,” he said.
To elicit an effective immune response against cancer is particularly challenging because the cancer is expecting an immune response
—Eli Gilboa, University of Miami
Coupling vaccination with existing chemotherapy or immunotherapy, such as checkpoint inhibitors, to circumvent the tumor microenvironment also offers a solution to break through tumor defenses.27-29 However, the antigen still must be displayed for T cells to engage and eliminate the tumor cells, highlighting the importance of restarting MHC-I surface presentation, which is interrupted in tumor cells at times due to mutations.30 If the MHC-I gene is mutated, this may be resolved by introducing an intact MHC-I copy using a vector.31 Often though, MHC-I gene expression is only downregulated and the gene is intact, so stimulatory cytokines could be sufficient to restart expression.32 Another idea demonstrated by Schliehe’s group is to use small molecules called proteolysis-targeting chimeras (PROTAC), which fuse target proteins to a protein tag for cellular degradation and in turn, promote MHC-I expression.33 In tumors, these could force cells to present these antigen-bearing complexes and the mutated antigens bound to them, which can improve vaccination success.
Better identification and selection of target antigens will also continue to propel cancer vaccines forward. Advancements in sequencing and predictive algorithms to find and validate novel tumor antigens in cancers could improve the outcomes of clinical trials by targeting antigens most likely to induce successful immune responses.34
Finally, giving cancer vaccines a twenty-first century makeover may make them not only more successful, but also more accessible. Crosby and her group replaced dendritic cells with viral platforms that introduce the HER2 antigen to dendritic cells in vivo.16,17 This approach can alleviate the complications surrounding isolating, preparing, and then readministering antigen-loaded dendritic cells to patients. Furthermore, ongoing research on neoantigens, particularly shared antigens across different tumors in various patients, can improve personalized cancer vaccines and help develop widely applicable therapeutic vaccines for patients.35
After almost fifty years of hope and promise, cancer vaccines are poised to join the repertoire of immunotherapies. Alongside existing therapies, cancer vaccines may produce immune responses that make tumors more accessible, revitalize the immune response against them, and improve the actions of other regimens to target and destroy cancerous cells. However, as Gilboa explained, battling cancer is a series of stepwise successes in improving patients’ lives. “We need patience and humility in recognizing the enormous challenge in treating cancer,” he said.
- Mukherji B, et al. Induction of antigen-specific cytolytic T cells in situ in human melanoma by immunization with synthetic peptide-pulsed autologous antigen presenting cells. Proc Natl Acad Sci. 1995;92(17):8078-8082
- Hollingsworth RE, Jansen K. Turning the corner on therapeutic cancer vaccines. NPJ Vaccines. 2019;4:7
- Salmon SE. Immunotherapy of cancer: Present status of trials in man. Cancer Res. 1977;37(4):1245-1248
- Hanna Jr MG, Peters LC. Immunotherapy of established micrometastases with Bacillus Calmette-Guérin tumor cell vaccine. Cancer Res. 1978;38(1):204-209
- Steinman RM, et al. Identification of a novel cell type in peripheral lymphoid organs of mice. V. Purification of spleen dendritic cells, new surface markers, and maintenance in vitro. J Exp Med. 1979;149(1):1-16
- Inaba K, et al. Dendritic cells pulsed with protein antigens in vitro can prime antigen-specific, MHC-restricted T cells in situ. J Exp Med. 1990;172(2):631-640
- Steinman RM, Witmer MD. Lymphoid dendritic cells are potent stimulators of the primary mixed leukocyte reaction in mice. Proc Natl Acad Sci. 1978;75(10):5132-5136
- van der Bruggen P, et al. A gene encoding an antigen recognized by cytolytic T lymphocytes on a human melanoma. Science. 1991;254(5038):1643-1647
- Monach PA, et al. A unique tumor antigen produced by a single amino acid substitution. Immunity. 1995;2:45-59
- Yu JS, et al. Vaccination with tumor lysate-pulsed dendritic cells elicits antigen-specific, cytotoxic T-cells in patients with malignant glioma. Cancer Res. 2004;64(14):4973-4979
- Avigan DE, et al. Phase I/II study of vaccination with electrofused allogeneic dendritic cells/autologous tumor-derived cells in patients with stage IV renal cell carcinoma. J Immunother. 2007;30(7):749-761
- Nestle FO, et al. Vaccination of melanoma patients with peptide-or tumorlysate-pulsed dendritic cells. Nat Med. 1998;4:328-332
- Boczkowski D, et al. Dendritic cells pulsed with RNA are potent antigen-presenting cells in vitro and in vivo. J Exp Med. 1996;184(2):465-472
- Heiser A, et al. Autologous dendritic cells transfected with prostate-specific RNA stimulate CTL responses against metastatic prostate tumors. J Clin Invest. 2002;109(3):409-417
- Hueman MT, et al. Analysis of naïve and memory CD4 and CD8 T cell populations in breast cancer patients receiving a HER2/neu peptide (E75) and GM-CSF vaccine. Cancer Immunol Immunother. 2007;56:135-146
- Crosby EJ, et al. Vaccine-induced memory CD8+ T cells provide clinical benefit in HER2 expressing breast cancer: A mouse to human translational study. Clin Cancer Res. 2019;25(9):2725-2736
- Crosby EJ, et al. Stimulation of oncogene-specific tumor-infiltrating T cells through combined vaccine and αPD-1 enable sustained antitumor responses against established HER2 breast cancer. Clin Cancer Res. 2020;26(17):4670-4681
- Carreno BM, et al. A dendritic cell vaccine increases the breadth and diversity of melanoma neoantigen-specific T cells. Science. 2015;348(6236):803-808
- Kantoff PW, et al. Sipuleucel-T immunotherapy for castration-resistant prostate cancer. N Engl J Med. 2010;363(5):411-422
- Bol KF, et al. Dendritic cell-based immunotherapy: State of the art and beyond. Clin Cancer Res. 2016;22(8):1897-1906
- Lesterhuis WJ, et al. Dendritic cell vaccines in melanoma: From promise to proof? Crit Rev Oncol Hematol. 2008;66(2):118-134
- Decker WK, et al. Dendritic cell immunotherapy for the treatment of neoplastic disease. Transplant Cell Ther. 2006;12(2):113-125
- Decker WK, et al. Cancer immunotherapy: Historical perspective of a clinical revolution and emerging preclinical animal models. Front Immunol. 2017;8:829
- de Grujil TD, et al. Whole-cell cancer vaccination: from autologous to allogeneic tumor-and dendritic cell-based vaccines. Cancer Immunol Immunother. 2008;57:1569-1577
- Sharma A, et al. HER-2 pulsed dendritic cell vaccine can eliminate HER-2 expression and impact ductal carcinoma in situ. Cancer. 2012;118(17):4353-4362
- Ramamoorthi G, et al. Intratumoral delivery of dendritic cells plus anti-HER2 therapy triggers both robust systemic antitumor immunity and complete regression in HER2 mammary carcinoma. J Immunother Cancer. 2022;10:e004841
- Ishikawa E, et al. Phase I/IIa of fracitionated radiotherapy, temozolomide, and autologous formalin-fixed tumor vaccine for newly diagnosed glioblastoma. 2014;121(3):543-553
- Le, DT, et al. Evaluation of Ipilimumab in combination with allogeneic pancreatic tumo cells transfected with a GM-CSF gene in previously treated pancreatic cancer. J Immunother. 2013;36(7):382-389
- Ott PA, et al. A phase Ib trial of personalized neoantigen therapy plus anti-PD-1 in patients with advanced melanoma, non-small cell lung cancer, or bladder cancer. Cell. 2020;183(2):347-362
- Garrido F, et al. The urgent need to recover MHC class I in cancers for effective immunotherapy. Curr Opin Immunol. 2016;39:44-51
- Del Campo AB, et al. Efficient recovery of HLA class I expression in human tumor cells after beta2-microglobulin gene transfer using adenoviral vector: Implications for cancer immunotherapy. Scand J Immunol. 2009;70(2): 125-135
- Seliger B. Novel insights into the molecular mechanisms of HLA class I abnormalities. Cancer Immunol Immunother. 2011;61:249-254
- Moser SC, et al. Acute pharmacologic degradation of a stable antigen enhances its direct presentation on MHC class I molecules. Front Immunol. 2018;8:1920
- Morse MA, et al. Vaccine therapies for cancer: Then and Now. Target Oncol. 2021;16:121-152.
- Katsikis PD, et al. Challenges in developing personalized neoantigen cancer vaccines. Nat Rev Immunol. 2023:1-15