ABOVE: Blackiston and his colleagues dovetailed biology and robotics to generate biobots derived from frog stem cells. These biobots can move due to cilia, small hairlike structures that cover their surfaces. Douglas Blackiston and Sam Kriegman, licensed under CC BY 4.0.
Douglas Blackiston, a developmental biologist at Tufts University, has always been fascinated by transformation. Using uncommon model organisms, from caterpillars and butterflies to tadpoles and frogs, he investigates how biology is adaptive. In one of his favorite projects, Blackiston transplanted eyes into the tails of blind tadpoles, restoring their vision in a striking display of tissue plasticity. This led him to an unusual spin-off project, where his work in biology dovetailed with robotics. In this work, Blackiston and his colleagues repurposed frog stem cells into programmable synthetic organisms to explore the design space of cells and their interactions.
What initially sparked your interest in insects, and how has that interest developed over time?
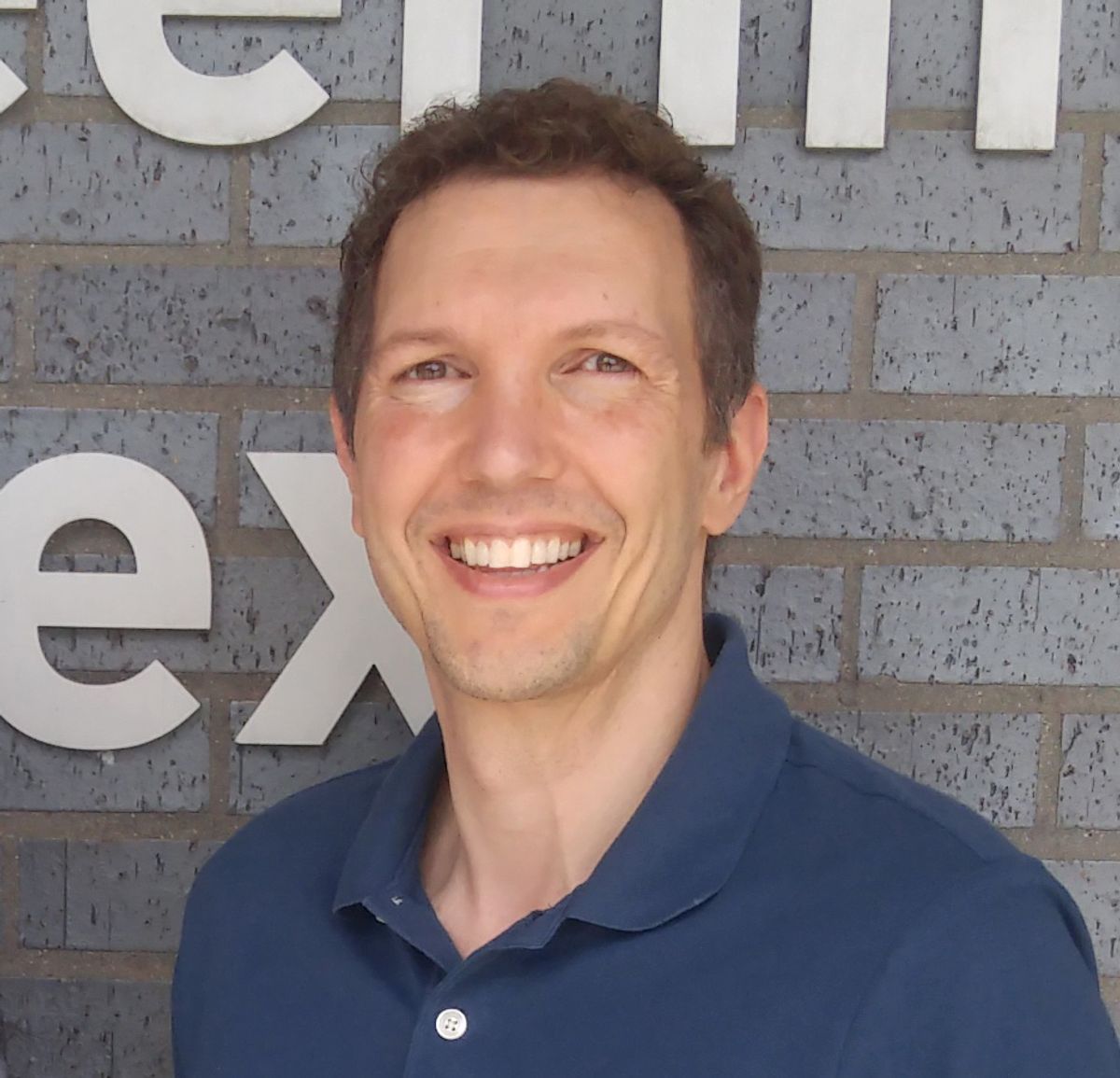
I grew up watching Transformers and loved things that transform, like toys and butterflies. I studied insects in college and graduate school, particularly caterpillars and memory. We wondered whether caterpillars could learn and retain information after undergoing complete metamorphosis into a butterfly or moth. As it turned out, caterpillar memories carried over into the moth stage.1
It’s remarkable how these basic caterpillars turn into creatures with compound eyes and sensitive sensory structures, enabling them to fly tens of thousands of miles during migration. I love this idea of nature doing something that crazy. However, conducting biomedically relevant studies with insects can prove challenging.
What makes the African clawed frog Xenopus laevis an attractive model organism for your research?
Frogs offered a nice middle ground when choosing model organisms for our studies. They are biomedically relevant as vertebrates, and we still get this cool transformation. While tadpoles also undergo metamorphosis, the contrast between tadpoles and frogs is even more pronounced. A tadpole breathes underwater, whereas a frog drowns underwater since it changed from having gills to lungs.
We use frogs because they share a large number of genes with humans, aiding our understanding of human disease.2 These frogs can live for about 20 years. A single female frog will lay approximately 2,000-5,000 eggs every three months for most of that time.
We have access to a huge amount of stem cell material. Since these eggs are externally fertilized in the water, we avoid invasive procedures to get the embryonic tissue. The eggs develop naturally in the water. After about 24 hours of development, we have 10,000-20,000 cells at our disposal.
Tadpole skin is shockingly like the inside of human lungs. By introducing human mutations for lung disease into frogs, they also have the same problems with their skin clearing mucus, mirroring human lung diseases. So, anything that this tissue does roughly translates into human airway disease and function. These studies are good opportunities to better understand the organization, repair, and function of lungs and airways.
How have you used tadpoles in your plasticity research?
I conducted experiments where I gave blind tadpoles eyes to restore their vision. I thought that was going to be my career and make me famous. A tadpole has a head and tail; its eyes are normally in its head. However, if a blind tadpole gets an eye back in its head, it will extend an optic nerve into the brain with a little bit of coaxing from biology.
One of the most interesting aspects of the project is a sort of validation because I can also put eyes at weird places like the tail.3 I wanted to show that even if I can grow eyes there, they don't connect effectively. When we transplant an eye on the tail, the eyes’ axons connect with either the spinal cord or gut. I was surprised to find that a huge number of these tadpoles can still see. This was because they sent optic nerves up into the spinal cord where they interfaced with something in a very odd way. The tadpoles can at least tell colors apart and patterns, but it's unclear how good the vision is, and I'm hoping to follow up with that in the future.4
Why don’t we just replace these cubes made out of silicone or fake materials with cells?
–Douglas Blackiston, Tufts University
It's this amazing plasticity that we can move these eyes around, and the brain will either figure out or learn to process the information dynamically. This process is sort of like a robot that has USB interfaces where we can unplug the camera and put it somewhere else. We're trying to understand the limits of how it happens and how it refines over time.
How did this work in tadpoles spin off into a project blending stem cells and robotics?
Since nature is good at building and rebuilding things, we want to put biology to work for humans, and we want biology to work with us. It was happenstance that a new project was born. We dovetailed two completely different disciplines: biology and robotics. The team consisted of myself and Michael Levin from Tufts University and roboticists Josh Bongard and Sam Kriegman from the University of Vermont. We used biology for all the work and robotics for all the theory to build biobots, also known as “xenobots.”5
Normally, roboticists build robots out of little silicone or other material cubes that change shape or just sit there. This got me thinking, “Why don’t we just replace these cubes made out of silicone or fake materials with cells?” In developmental biology and stem cell biology, this isn’t a super difficult trick. The technology exists. But no one's thought about doing this, and it’s like building an organism.
Roboticists build all the time, but for me as a biologist, it was an interesting frameshift. I used a computer that ran a simulation that looked like the video game Minecraft and interacted with it by simulating physics and friction. However, translating it into the real world is a major challenge.
A huge amount of excellent work has been done over the past 50 years to understand how to play with cells; we can turn them into neurons, skin, and heart cells. So, we stood on that diverse body of literature and essentially broke down this embryonic tissue from this frog. Then we made a palette of different building blocks, like Lego components, and reassembled them into whatever arbitrary shape or size we wanted based on the simulations.
Designing these biological robots helps lay the foundation for understanding what the system is capable of and then building. We have observed different behaviors such as aggregation and interlocking, and we hope to take this work to another level to learn more fundamental basic science.
How has this collaboration benefited you outside of research?
I've learned so much about other disciplines. There are things we can do with cells that roboticists say will never be possible with their materials, but it is possible for cells. While the roboticists thought the biology in this project was magic, I found the computer science aspect magical.
It’s hard for me to bring biology to classrooms and do outreach work because there are regulations around transporting animals and cells. However, simulations are incredible tools for education because they act as gateways that get kids to interact with us. We're trying to create outreach programs where students can either build artificial xenobots in the class or simulated ones.
We want to have local competitions where students send me the design, I build the designs in the laboratory, and we see if it works. It lets the kids participate in the design process. Although artificial intelligence and simulation can get a bad rap, there are some great opportunities to remove barriers to hypothesis testing and engaging with science, especially with the younger community.
This interview has been condensed and edited for clarity.
- Blackiston DJ, et al. Retention of memory through metamorphosis: can a moth remember what it learned as a caterpillar? PLoS One. 2008;3(3):e1736.
- Nenni MJ, et al. Xenbase: Facilitating the use of Xenopus to model human disease.Front Physiol. 2019;10:154.
- Blackiston DJ, Levin M. Ectopic eyes outside the head in Xenopus tadpoles provide sensory data for light-mediated learning. J Exp Biol. 2013;216(Pt 6):1031-40.
- Blackiston DJ, et al. Serotonergic stimulation induces nerve growth and promotes visual learning via posterior eye grafts in a vertebrate model of induced sensory plasticity. npj Regen Med. 2017;2:8.
- Kriegman S, et al. A scalable pipeline for designing reconfigurable organisms. Proc. Natl. Acad. Sci. U.S.A. 2020;117:1853–1859.