ABOVE: By using capsid protein engineering, scientists improve the specificity and potency of AAV-based gene delivery to the brain. Modified from ©istock.com, Jolygon; designed by Imran Chowdhury
The blood-brain barrier (BBB) is a cellular fortress that regulates what comes in and goes out of the brain. It ensures that the brain has access to key nutrients while protecting it from pathogens and toxins. On the flip side, the BBB blocks many promising therapeutics. However, system upgrades to the leading gene delivery vehicle offer hope that effective therapies will soon be within reach.
Although researchers are investigating lipid nanoparticles and other delivery vehicles, adeno-associated virus (AAV) vectors remain the leading in vivo gene therapy delivery platform due to their unmatched targeting capabilities, lasting expression in nondividing cells, and lack of pathogenicity in humans. “It’s safe to say that if we can get the AAV there, then it’s a one and done therapy,” said Beverly Davidson, a gene and cell therapy researcher at the University of Pennsylvania.
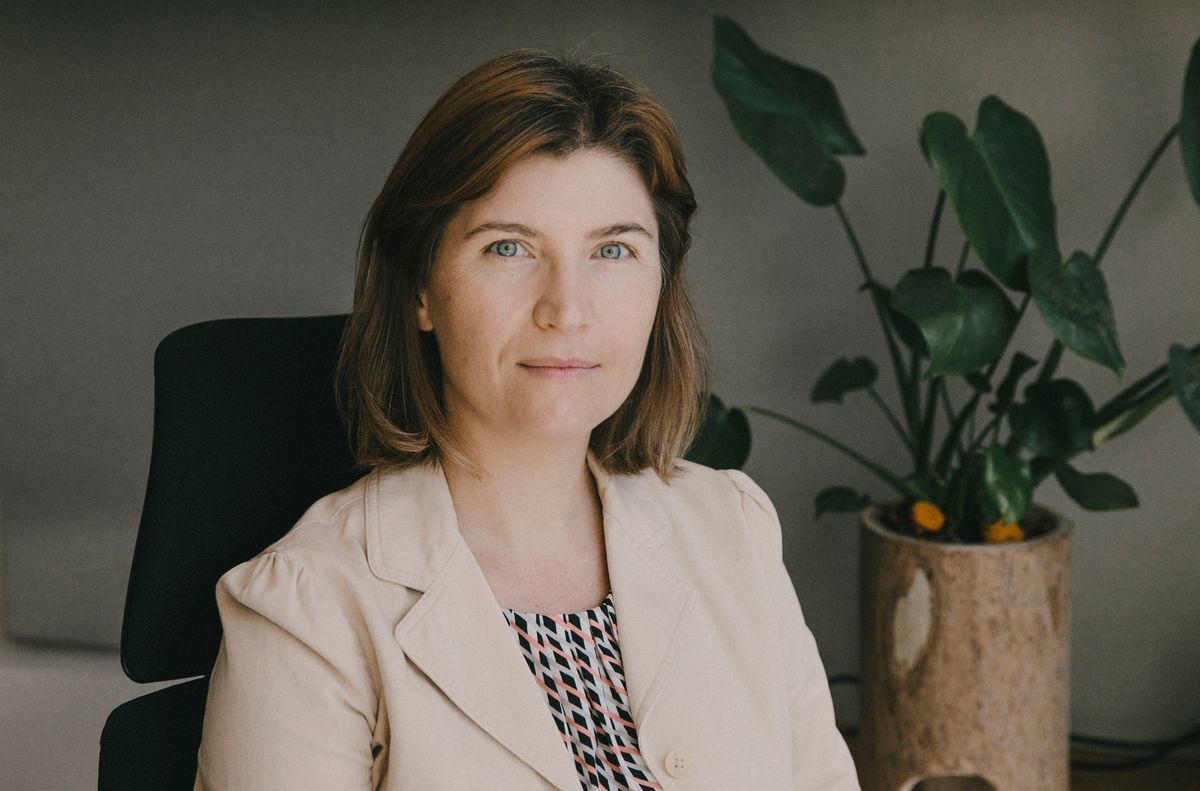
There is a growing list of approved AAV-based gene therapies and hundreds more in the clinical pipeline. However, many of these therapies developed to treat CNS disorders require invasive measures, including direct injections into the brain or lumbar punctures, which can also limit the therapy’s reach in the brain. “[They] have poor spatial distribution, but they are done out of great necessity to bypass the blood brain barrier,” said Viviana Gradinaru, a neuroscientist at the California Institute of Technology (Caltech). Limited distribution may be acceptable if the goal is localized expression of the gene product, but many disorders have widespread effects in the brain.
“In theory, the vasculature would be the best delivery highway for the very simple reason that the human brain has about 400 miles of vasculature,” Gradinaru added. This extensive arterial network services the brain’s nearly 100 billion neurons, but to cruise down the brain’s vasculature highway, an AAV delivered intravenously must cross through the BBB’s border control.1
“If we learn the rules of this highly selective portal, we could benefit from that basic biology knowledge to access the brain with therapeutics,” said Gradinaru, who has developed AAV engineering strategies to drive gene delivery to the brain for the last decade, despite not knowing the rules of the road.
AAV9: Teacher and Template
The two basic elements of an AAV are the viral DNA and its protein shell, or capsid. To transform the virus into a gene therapy, scientists swap the viral DNA with new genetic cargo to manipulate gene expression. Although scientists have identified the genetic causes of many inherited diseases, they have struggled to safely deliver the precious DNA cargo that corrects the problem to patients. This relates partly to the capsid. In addition to protecting the viral genome, this icosahedral protein cage determines which tissues and cells the virus accesses via its interactions with cell surface receptors. Scientists have discovered 13 naturally occurring AAV serotypes, and although many infect brain cells with varying degrees of efficiency, AAV9 and the lesser-studied AAVrh.10 are the only ones that cross the BBB.2,3
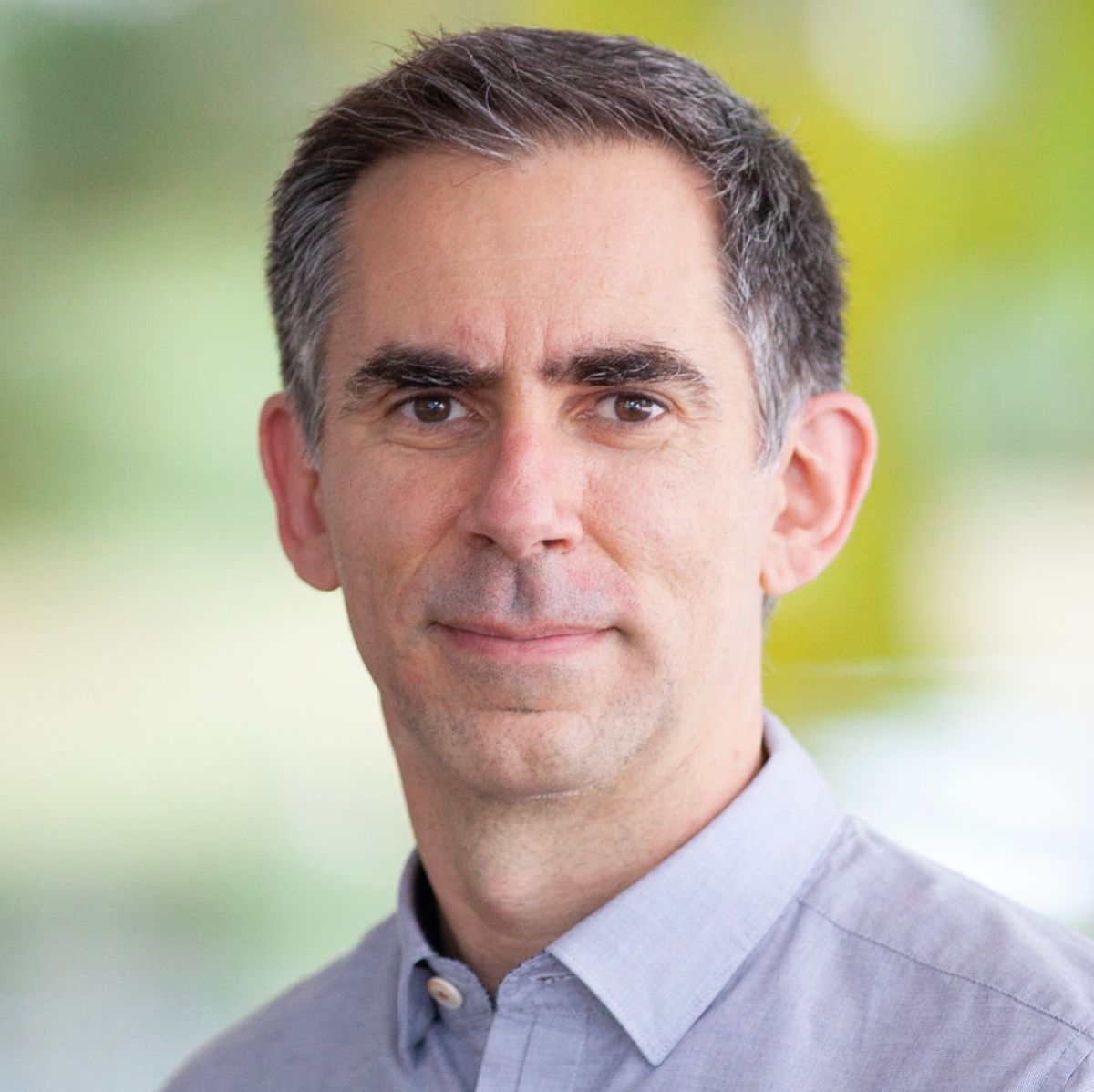
Zolgensma, an FDA-approved AAV9 therapy that targets the root cause of spinal muscular atrophy, exhibited incredible success in children under the age of two. However, AAV9 wasn’t the one-size fits all platform for CNS-targeted gene therapies that scientists had hoped for. Changes in brain development are accompanied by changes in the virus’s proclivity for different cell types. One nonclinical study showed that although the virus crossed the BBB, it preferentially targeted neurons in neonatal mice and non-neuronal astrocytes in adult mice.4
Given these changes, the same dose in an adult would target only a fraction of the brain’s neurons, but increasing the dose would push against safety limits. Of particular concern is the virus’s liking for the liver. “If you look at the number of vector genomes you're delivering to an individual liver hepatocyte at that dose, it's in the range of hundreds of viral copies per cell, but you're delivering, on average, much less than one viral genome per cell to the brain,” said Ben Deverman, a molecular biologist at the Broad Institute.
To flip the ratio and bias an AAV away from toxic hotspots and towards a target organ or cell, researchers like Deverman, Gradinaru, and Davidson have developed capsid engineering strategies to put new AAV models on the production line.
Creating Capsids
There are two main approaches to capsid engineering. “If you know the rules, you engineer against that rule,” said Gradinaru. Using rational design, knowledge of a specific receptor on the BBB allows scientists to edit the capsid accordingly, but scientists have only identified a few of these portals. Over a decade ago, Gradinaru decided that without knowing the rules, the best way to design an AAV was through a mechanism agnostic approach. This engineering solution was inspired by conversations she had with her Caltech colleague Frances Arnold, who received a Nobel Prize for her work on directed evolution to engineer enzymes.
“What we learned from those conversations was that directed evolution can be the solution to this problem because you do not need to know the biological rules to make progress,” said Gradinaru, with the caveat that there is a good teacher template and stringent selection criteria in place. As a starting point, Gradinaru and her team turned to AAV9. “Why not start with something that has the property you want, but now you redirect it and tweak it a little bit so that it does so more efficiently,” said Davidson.
When a pathogen interacts with a host, it evolves to improve its virulence, targeting abilities, and survival. With a high number of hosts, the pathogen has more opportunities to evolve, as evident from the continued emergence of new SARS-CoV-2 variants. “This is exactly the lessons that we use for the AAV9 work,” said Gradinaru. Using directed evolution, researchers found that they could speed up the evolutionary timeline by repeatedly generating, testing, and selecting for viral vectors that exhibited specific characteristics, such as enhanced targeting abilities.5
In the early 2010s, Deverman joined Gradinaru’s team at Caltech as a postdoctoral researcher, where he developed a pipeline to create and test novel AAV.6 To generate a library of new capsids, Deverman edited a section of the AAV genome that contained a stretch of seven amino acids that he found can tolerate a bit of change but still form a capsid. By editing this tiny bit of viral real estate, Deverman and his colleagues produced many capsids to test. With protein engineering, “The number of possibilities is more than we can ever possibly test,” said Deverman. “Even with a protein the size of an AAV there're more possibilities than there are stars in our universe. Most of those can’t make a capsid, but that’s still too many to test in a lifetime.”
A seemingly never-ending list of possible capsid candidates demanded a stringent test that selects those that most efficiently deliver genetic cargo to the brain. To specifically select for viruses that successfully traversed the BBB, Deverman injected Cre transgenic mice with his capsid library, isolated DNA from their brain tissues, and then sequenced the genomes of the viruses that successfully infected the Cre-expressing cells. He then used these recovered sequences to generate a second library for another round of testing. This pipeline resulted in several viral variants, but Deverman and his team decided to advance the most successful variant, which they called AAV-PHP.B, for a head-to-head comparison with AAV9. For this experiment, they loaded green fluorescent protein (GFP) into each virus, administered them to mice intravenously, and compared delivery efficiencies of the GFP molecules across the brain. AAV-PHP.B showed at least 40-fold higher gene transfer than the standard AAV9.
“This kind of got everybody very interested in what's possible because it really showed that you can enhance the function of these capsids by 100-fold or more relative to the natural serotypes,” said Deverman. Others have gone on to develop engineered AAV with 400-fold higher gene transfer to the brain.7
“That was basically the first chapter of a manual of how to engineer AAV without knowing the mechanism,” said Gradinaru, who has gone on to write several other chapters in the AAV engineering book. However, translational complications soon cropped up.
Species Specificity
As other researchers started using this new capsid, an important discovery came to light. Experiments in nonhuman primates (NHP) like marmosets and rhesus macaques using AAV-PHP.B showed limited delivery efficiency, suggesting potential species differences in how the capsid interacts with the BBB.8,9 Furthermore, not only was the capsid's remarkable efficiency seemingly specific to mice, it was specific to the C57BL/6J strain.10
Deverman’s team and others leveraged these differences to probe the mechanism by which the engineered AAV crossed the BBB in that mouse strain and discovered a new receptor, lymphocyte antigen 6 family member A (LY6A).11,12 Thus, species that lack a functional LY6A will exhibit limited, if any, expression of AAV-PHP.B cargo.
Given their evolutionary proximity to humans, NHP are ideal models for testing therapies. However, the scarcity and cost of using NHP requires a careful and considered selection process. When Gradinaru started to extend viral vector validation to NHP, she still relied on directed evolution of AAV in mice.13 In one study, Gradinaru and her team generated a pool of mouse-evolved viruses and found that some variants showed improved brain targeting capabilities in rats, which diverged from humans 75 million years ago, and marmosets, New World monkeys that split off only 40 million years ago.14
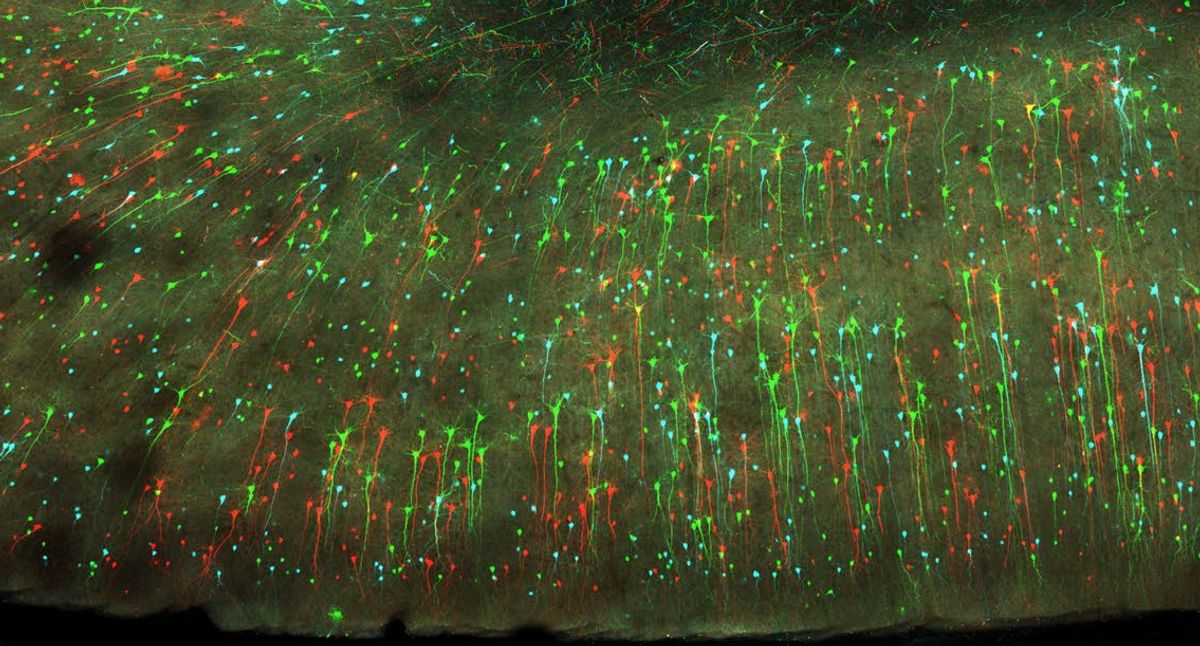
To cut out the middle mice and go straight from generating a library to testing the engineered variants in NHP, Gradinaru needed a new approach.15 A similar Cre-driven NHP system to generate selection pressure wasn’t possible as Cre-transgenic marmosets are currently unavailable.16 So her team used next-generation sequencing data collected from variants that successfully crossed into the brain to generate network graphs. Instead of advancing only the most successful capsids, which could be biased towards a single mechanism of entering the brain that is specific to marmosets, they selected a variety of AAV signatures for a second round of testing in newborn rhesus macaques, Old World monkeys that diverged from humans 25 million years ago.
AAV.CAP-Mac came out on top, so they advanced this variant for further testing in multiple primate species and in cultured human neurons, where it exhibited improved gene delivery efficiency throughout the brain. However, differences still emerged in the data. “I was hoping for higher similarities across all of these different biological settings, [but] we have to take the experiments for what they tell us,” said Gradinaru. For example, CAP-Mac was biased towards vasculature in the adult marmoset and neurons in infant macaques, echoing previous studies that revealed age differences within a species.
These studies in NHP advanced AAV testing closer to humans and also demonstrated that it’s possible to preferentially steer AAV towards the brain. By changing just one or two amino acids in the peptide sequences, Gradinaru’s team biased the AAV towards endothelial cells or neurons and away from the liver.17,18 By increasing the specificity, researchers can lower the dose and reduce off-target toxicities.
“The real bar, in my opinion, is to completely detarget these from peripheral organs systems. If you're going to put these high dose capsules into animals and people for brain directed therapies, then we need to minimize the risk to the peripheral tissues,” said Davidson.
The Rules of the Road
By following a mechanism agnostic approach, scientists have generated thousands of new AAV exhibiting different gene delivery signatures throughout the body. “We can use these AAV libraries to find the mechanism of action, and that's actually something that's an unprecedented opportunity in the field,” said Gradinaru.
Deverman described the discovery of the LY6A portal into the mouse brain as a turning point for his team. “It may seem a little bit obvious when you think about the fact that we engineered an AAV that was more than 100-fold more efficient than AAV9 at crossing the BBB that it probably did something new, but we didn’t know what that meant,” he said. Before these findings, they weren’t sure whether they had engineered a capsid that used the same receptor as AAV9, but just used it better, or whether they engineered a capsid that could bind a completely different receptor. LY6A’s discovery suggested to them that they could test the ability of an engineered AAV to bind specific known proteins prior to graduating the capsids to time-consuming and costly in vivo experiments.
Deverman and his team recently transformed that idea into a high-throughput protein assay.19 To select for capsids that bind to LY6A, they mixed their large AAV library with a slurry of magnetic beads attached to the purified protein and pulled out capsids that stuck to the beads. As expected, among the positive hits, they identified representatives of the AAV-PHP.B family. Deverman predicted that they could use this approach to develop capsids from a novel target. To test this, they developed another protein bead potion for LY6 family member C1 (LY6C1), which is uniformly expressed across all mouse strains, and discovered thousands of sequences that bound LY6C1. When they tested these in mice, hundreds of capsids successfully entered the brain to infect neurons and glial cells. “This was great; it really exceeded our expectations,” said Deverman.
This approach is also more sensitive. With in vivo screens, researchers throw thousands of capsid variants at a single brain and then recover the capsids that make it through. However, Deverman noted that, at this stage, the library is too diverse, and recovering each AAV is difficult, which can limit reproducibility. “If you put the same library into three mice, you will get 10,000 unique sequences out of each mouse and very little overlap because you're just sampling and grabbing from tens of millions of capsids,” said Deverman.
With their updated approach, they consistently recovered capsids with greater diversity and then used these sequences to train generative machine learning models to predict even more sequences that should have the same capabilities. “By the time we go into a second round of screening, we can already be maturing the sequences and exploring even more than what we found in the first round,” said Deverman.
Gradinaru also uses new computational and protein structure prediction tools to uncover novel AAV. When probing the mechanisms by which certain AAV variants with enhanced brain expression cross the BBB, two targets popped out: the already identified LY6C1 and a more unusual avenue, the enzyme carbonic anhydrase IV (CA-IV).20 “It's an enzyme we have on our tongue to feel sparkling soda,” said Gradinaru.21 “It's also an enzyme that's extremely abundant on the blood brain barrier,” where it regulates blood pH.22 Furthermore, it’s conserved. “Mice have it; we have it,” said Gradinaru.
While some sites on the enzyme are completely conserved, others have significant sequence modifications, so depending on where an engineered AAV binds, it can have similar or distinct effects across species. To advance their target-driven approach to engineering AAV, Gradinaru’s team turned to AlphaFold, an artificial intelligence based protein folding predictor.23 For this, they fed AlphaFold the CA-IV binding pocket along with peptides found in engineered AAV. “AlphaFold tells us which ones will work in rodents, in macaques, and this is big, in humans without testing in humans,” said Gradinaru.
Given the potential for species-specificity, AAV evolved in NHP may not work well in humans. Approaches like Gradinaru’s AlphaFold predictor and Deverman’s pull-down assay may inform the development of more human-specific gene delivery vectors and biologics that can cross the brain. In a recent preprint posted on bioRxiv, Deverman and his team reported using their receptor-targeting in vitro screen to discover AAV variants that bind the human transferrin receptor, a protein expressed on the BBB.24
As scientists learn more about the brain vasculature in health and disease, this potentially provides new targets for a mechanism focused selection of novel AAV capsids. Additionally, getting into the brain is just one hurdle.25,26 As highlighted in the recent suite of papers on a comprehensive cell atlas of the human adult and developing brain, the brain is home to a rich diversity of cells.27,28 By further engineering the surfaces of the capsids that cross the BBB against various targeting needs, scientists could achieve even greater specificity once the capsids travel inside the brain.
“The field is going to learn a lot over the next few years about what types of receptors are capable of mediating AAV transcytosis, and that's going to just come from testing various hypotheses,” said Deverman. “I'm really enthusiastic about it. I feel like we're right on the cusp of making some major progress.”
Many brain diseases for which there are few treatments, including neurodegenerative, neuromuscular, and lysosomal storage diseases, could benefit from advancements in vector engineering.
“The field has moved into more sophisticated ways of designing, testing, and applying AAV for gene therapies. In the next five to seven years, we'll really see the application of these come to fruition,” said Davidson.
Viviana Gradinaru is a cofounder and board member of Capsida Biotherapeutics and has a patent for her AAV engineering research described in this story.
Ben Deverman is a scientific founder and scientific advisory board member at Apertura Gene Therapy and a scientific advisory board member at Tevard Biosciences. He has filed a patent related to the research in Huang Q, et al. bioRxiv, 2023.
- Zlokovic B, Apuzzo, M. Strategies to circumvent vascular barriers of the central nervous system. Neurosurgery. 1998;43(4):877-878.
- Srivastava A. In vivo tissue-tropism of adeno-associated viral vectors. Curr Opin Virol. 2016;21:75-80.
- Wang D, et al. Adeno-associated virus vector as a platform for gene therapy delivery. Nat Rev Drug Discov. 2019;18(5):358-378.
- Foust KD, et al. Intravascular AAV9 preferentially targets neonatal neurons and adult astrocytes. Nat Biotechnol. 2008;27(1):59-65.
- Maheshri N, et al. Directed evolution of adeno-associated virus yields enhanced gene delivery vectors. Nat Biotechnol. 2006;24(2):198-204.
- Deverman BE, et al. Cre-dependent selection yields AAV variants for widespread gene transfer to the adult brain. Nat Biotechnol. 2016;34(2):204-209.
- Nonnenmacher M, et al. Rapid evolution of blood-brain-barrier-penetrating AAV capsids by RNA-driven biopanning. Mol Ther Methods Clin Dev. 2020;20:366-378.
- Matsuzaki Y, et al. Intravenous administration of the adeno-associated virus-PHP.B capsid fails to upregulate transduction efficiency in the marmoset brain. Neurosci Lett. 2018;665:182-188.
- Liguore WA, et al. AAV-PHP.B administration results in a differential patterns of CNS biodistribution on non-human primates compared with mice. Mol Ther. 2019;27(11):2018-2037.
- Hordeaux J, et al. The neurotropic properties of AAV-PHP.B are limited to C57BL/6J mice. Mol Ther. 2018;26(3):664-668.
- Huang Q, et al. Delivering genes across the blood-brain barrier: LY6A, a novel cellular receptor for AAV-PHP.B capsids. PLoS One. 2019;14(11):e0225206.
- Hordeaux J, et al. The GPI-linked protein LY6A drives AAV-PHP.B transport across the blood-brain barrier. Mol Ther. 2019;27(5):912-921.
- Chen X, et al. Engineered AAVs for non-invasive gene delivery to rodent and non-human primate nervous systems. Neuron. 2022;110(14):2242-2257.
- Goertsen D, et al. AAV capsid variants with brain-wide transgene expression and decreased liver targeting after intravenous delivery in mouse and marmoset. Nat Neuro. 2022;25(1):106-115.
- Chuapoco MR, et al. Adeno-associated viral vectors for functional intravenous gene transfer throughout the non-human primate brain. Nat Nanotechnol. 2023;18(10):1241-1251.
- Okano H, et al. Brain/MINDS: A Japanese national brain project for marmoset neuroscience. Neuron. 2016;92(3):582-590.
- Chen X, et al. Functional gene delivery to and across brain vasculature of systemic AAVs with endothelial-specific tropism in rodents and broad tropism in primates. Nat Commun. 2023;14(1):3345.
- Goertsen D, et al. AAV capsid variants with brain-wide transgene expression and decreased liver targeting after intravenous delivery in mouse and marmoset. Nat Neurosci. 2022;25(1):106-115.
- Huang Q, et al. Targeting AAV vectors to the central nervous system by engineering capsid–receptor interactions that enable crossing of the blood–brain barrier. PLoS Biol. 2023;21(7):e3002112.
- Shay TF, et al. Primate-conserved carbonic anhydrase IV and murine-restricted LY6C1 enable blood-brain barrier crossing by engineered viral vectors. Sci Adv. 2023;9(16):eadg6618.
- Chandrashekar J, et al. The taste of carbonation. Science. 326(5951):443-445.
- Ghandour MS, et al. Carbonic anhydrase IV on brain capillary endotherlial cells: A marker associated with the blood-brain barrier. Proc Natl Acad Sci USA. 1992;89(15):6823-6827.
- Jumper J, et al. Highly accurate protein structure prediction with AlphaFold. Nature. 2021;596(7873):583-589.
- Huang Q, et al. An AAV capsid reprogrammed to bind human Transferrin Receptor mediates brain-wide gene delivery. bioRxiv. 2023;2023.12.20.572615.
- Winkler EA, et al. A single-cell atlas of the normal and malformed human brain vasculature. Science. 2022;375(6584):eabi7377.
- Sun N, et al. Single-nucleus multiregion transcriptomic analysis of brain vasculature in Alzheimer’s disease. Nat Neurosci. 2023;26(6):970-982.
- Velmeshev D, et al. Single-cell analysis of prenatal and postnatal human cortical development. Science. 2023;382(6667):eadf0834.
- Siletti K, et al. Transcriptomic diversity of cell types across the adult human brain. Science. 2023;382(6667):eadd7046.